Observations Pallav Kosuri Making magic out of molecules
Salk Assistant Professor Pallav Kosuri’s work sounds like it’s straight out of science fiction. A physicist-turned-bioengineer, Kosuri is developing nanoscale technologies that are on their way to transforming how we diagnose and treat diseases. But it’s his choice of building material that is especially surprising—these mini-machines are made almost entirely out of DNA.
Inside Salk sat down with Kosuri to hear more about his DNA designs and how they’re bringing biology and medicine into the future.
Kosuri’s lab is using DNA to create a suite of biosensors, diagnostic tools, and drug delivery systems. He’s even teamed up with NASA-engineer-turned-YouTuber Mark Rober to create the world’s smallest NERF gun—a microscopic device modeled after a real toy gun, just three million times smaller. (Versatility is clearly a strong suit of the technique.)
When did you first become interested in science?
PK: I was one of those kids who devoured books—encyclopedias, science fiction, fantasy, anything I could find. Science fiction was particularly magical for me. I loved the idea of alien technologies and discovering new worlds. At some point, it hit me that the “magic” of science fiction wasn’t entirely fiction. The world around us—whether it’s biology, physics, or technology—already operates like magic, and the key to understanding this magic is science.
That drive to “understand the magic” shaped everything. In high school, I thought I’d end up building rockets or particle accelerators because physics seemed like the ultimate way to explore the unknown. I was so inspired by the golden age of physics when scientists like Ernest Rutherford or Marie Curie could have an idea and conduct their own experiments, and their personal findings could change the course of history.
But over time, I came to see that I was actually living in a different golden age. These days nearly all the most transformative discoveries are happening in biology. A scientist can use a new tool and suddenly reveal something that totally changes the way we think about the nature of life. I realized I could make a much bigger impact if I applied my engineering skills to these questions in biology, and it’s been an incredibly exciting journey ever since.
What part of your training most inspired the work you do today?
PK: It really started during my PhD. I came to the United States from Sweden as a Fulbright Scholar to work in biochemistry and biophysics, but after years of studying lasers and subatomic particles, I had a lot of biology to catch up on. Thankfully, I found an incredible mentor at Columbia University, Professor Julio Fernandez, who helped me find my footing in this new field between physics and biology.
Together, we studied how a spring-like protein called titin helps our muscles stretch and contract. To study this protein, we built a new kind of atomic force microscope that could measure and apply forces to a single molecule. Using this ultra-precise instrument, I could grab an individual titin molecule and measure its stiffness under different conditions. To my surprise, we discovered that certain chemical changes have a strong effect on titin’s stiffness. What’s more, changing the stiffness of this one protein could change the overall stiffness of the entire muscle. This discovery turned out to be very important for understanding how muscles work and how we can tune their mechanics. We can now use this information to study muscle conditions such as heart disease or design better robotics and artificial limbs.
It was fascinating work, but while I was engineering these molecule-manipulating microscopes, I kept thinking, Why are we building such massive machines to study something so small? If we want to study molecules, why don’t we build tools at the molecular scale? That question led me straight into the world of nanotechnology.
Why is nanotechnology so important?
PK: We’ve made huge advances in computer science by shrinking electrical circuits down to the microscopic level and then to the nanoscale. That’s why our smartphones and other devices are so much smaller, faster, and more powerful. It’s inevitable that nanotechnology will have a similar impact on biology. It’s the direction that a lot of bioengineering is headed in.
In biology, nanotechnology lets us design devices that work at the same scale as the molecules we’re trying to study and manipulate. Our cells are made of tiny molecular machines that work together in incredibly precise ways. If we want to truly understand biology or develop new medical solutions, we need tools that work at that scale. In the end, these new tools will also be much faster, cheaper, and more accessible than our current technologies.
How do you build machines out of DNA?
PK: DNA might seem like an unusual choice, but it’s an incredible material for this purpose. It’s relatively simple in structure yet highly programmable and very precise in its assembly. It’s also 100 percent biocompatible and nontoxic. We use a technique called DNA origami to fold the strands into different shapes. Think of it like molecular LEGO. DNA is the building block, and we can design it to self-assemble into specific structures that carry out important functions with high efficiency.
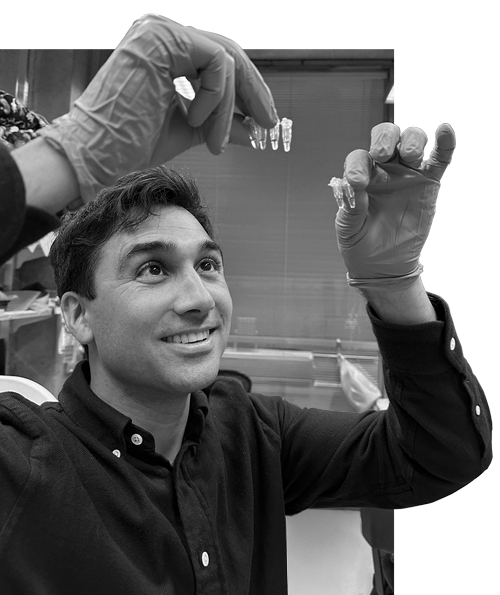
“What excites me most is the unknown. When you start working on this scale—building devices out of molecules—you’re opening an entirely new frontier of engineering.”
–Assistant Professor Pallav Kosuri
How will this technology help advance health and medicine?
PK: There are so many potential applications, but I can share a few that we’re especially excited about.
My lab is currently developing a range of DNA-based nanosensors, which are tiny devices that can detect molecules, electrical currents, physical forces—all sorts of things that scientists want to measure. They’re super sensitive and extremely precise, so they have the potential to advance a lot of the research here at Salk.
For example, we know that nearly half of all adults in the US have high blood pressure, but how exactly does this pressure affect the heart? Which cells are most vulnerable to being damaged by this mechanical stress, and how can this lead to heart disease? To study this, we’re developing a nanosensor that glows when it senses a certain amount of pressure. Our vision is to use such sensors to create a detailed map of how mechanical stress is distributed across the heart. Being able to measure these aspects of biology will help us better understand and treat many different diseases.
What’s even more exciting is that these kinds of nanosensors can also be used as diagnostic tools. Imagine going to the doctor, and instead of having multiple vials of blood drawn and waiting days for the results, the clinicians only need to take a tiny sample and can analyze it instantly. And they’re not just doing 1 or 2 or 10 different tests that the doctor specifically ordered. They’re using thousands of different sensors at a time to give you a much fuller picture of your health. Most experts in the field of molecular diagnostics agree that this will be possible at some point in our future; my personal conviction is that DNA sensors will be the fastest way to get there.
But most importantly, because DNA-based sensors can detect single molecules, they can spot a disease before symptoms even appear. For example, a nanosensor would only need to find a single piece of viral RNA to detect an infection and could even identify the specific strain. This has huge implications, especially for cancer, for which early detection is so critical. If we could catch cancers when they’re still just a few cells, we could dramatically improve treatment outcomes and save millions of lives.
We could also potentially use DNA origami to design better therapeutics. Instead of flooding the patient’s body with a systemic drug, we could develop tiny drug-delivery systems that bring the medication directly to the specific cells that need it. This level of precision could make treatments more effective while avoiding all the unwanted side effects that can come with traditional therapies.
What excites you most about DNA origami?
PK: I think we’re on the verge of a revolution in molecular diagnostics and medicine. The tools we’re building with DNA origami will help transform how we detect and treat diseases. But beyond these clear applications, what excites me most is the unknown. When you start working on this scale—building devices out of molecules—you’re opening an entirely new frontier of engineering. I mean, who would have thought that studying molecular mechanics would lead us to develop better cancer diagnostics? To paraphrase Jonas Salk, the possibilities are only limited by our imagination and our ability to work hard to turn those dreams into reality.
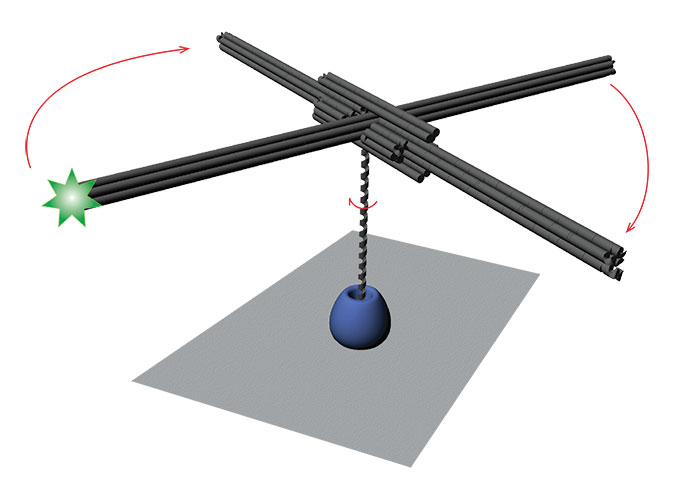
Why is Salk the right place for you to pursue this work?
PK: What really sets Salk apart is its focus on big, bold science. Everyone here is driven by Jonas Salk’s vision of being “good ancestors.” We all want to leave a legacy of discovery that truly benefits future generations. That ethos allows us to pursue high-risk, high-reward ideas—like using DNA to build molecular machines—that might not fit into traditional academic settings or receive sufficient federal funding.
Salk is also a unique institute because it doesn’t confine researchers to a single discipline. Here, we don’t have departments—we’re encouraged to collaborate across fields. That’s critical for my work because it sits at the intersection of biology, physics, and engineering. I can walk down the hall and talk to a cancer biologist, an immunologist, or a neuroscientist, and each conversation sparks new ideas and directions that no individual field could achieve alone.
What we’ve all come to recognize is that the biggest advances we can make stem from the cross-pollination of different disciplines, where everyone brings something to the table and the result is much greater than the sum of the parts.
It’s just like biology, really. Most of nature is made of just four elements: oxygen, carbon, hydrogen, and nitrogen. You have these few well-defined things, but when you mix them together in the right way, the complexity that arises is beyond our wildest imagination.
It’s magic—and that’s what inspires my work here at Salk.
Worlds smallest Nerf gun shoots an ant
In September 2023, The Kosuri Lab at the Salk Institute collaborated with YouTuber@MarkRober to use DNA origami to make the world’s smallest NERF blaster. Click on the button below to watch the video that has been viewed over 70 million times on YouTube.
Featured Stories
Seeds of change: The Harnessing Plants Initiative is scaling a new kind of crop that could save the future of farming—and the planetFarmers and plant biologists are linking arms to build more sustainable, resilient agriculture. Salk scientists are working to enhance plants' natural ability to capture carbon to clean our air and restore environmental stability—all while maintaining productivity for growers.
The day polio met its match: Celebrating 70 years of the Salk vaccineSeventy years ago, on April 12, 1955, the polio vaccine developed by Jonas Salk and his colleagues was officially declared “safe, effective, and potent”—a moment heralded as a triumph of medicine over one of the most feared diseases of the 20th century. On this milestone anniversary, it's crucial to remember that fear, and learn from this historic public health success.
50 years of discovery: Professor Tony Hunter’s half-century legacy at SalkTony Hunter first arrived at the Salk Institute in 1971 as a postdoctoral trainee from the University of Cambridge. Four years later, he officially joined the Institute as an assistant professor and cancer biology pioneer.
Pallav Kosuri: Making magic out of moleculesA physicist-turned-bioengineer, Kosuri is developing nanoscale technologies that are on their way to transforming how we diagnose and treat diseases. Kosuri’s lab is using DNA to create a suite of biosensors, diagnostic tools, and drug delivery systems.
Suzanne Page: Uprooting, replanting, and blooming againIn October 2024, the Salk Institute named Suzanne Page as its new Vice President and Chief Operating Officer. Page has lived and traveled all over the country, developing a strong background in research operations, finance, and legal in the for-profit and nonprofit sectors—leading her to "manifest" her role at Salk.
Irene López Gutiérrez: After every storm comes sunshine—and scienceRainy winter weather in Gutiérrez's seaside hometown in Spain led to long days indoors, where she found a science television show that inspired an entire life of education and research that eventually brought her to Salk. Today, she works in Professor Susan Kaech's lab studying Alzheimer's disease.
Michelle Chamberlain named Salk’s new Vice President of External RelationsMichelle Chamberlain assumed the role on April 2, where she will serve on Salk's Executive Leadership Team and oversee all fundraising efforts, communications, community engagement, education outreach programs, foundation relations, and stewardship activities.
Trustee Richard A. Heyman donates $4.5 million to enable early-stage innovative researchRichard A. Heyman, a member of the Salk Institute’s Board of Trustees, and his wife, Anne Daigle, have donated $4.5 million to establish the new Richard A. Heyman Collaborative Innovation Fund to support Institute faculty on collaborative, early-stage studies aimed at big, bold questions.