Frontiers Connecting the dots—From the immune system to the brain and back again
Colleagues used to call marketing specialist Cynthia Adinig “the human computer.” She had a nearly photographic memory and was teaching her young son algebra and chess strategies. Then, in early 2020, she caught COVID-19. Initially, she had a mild case, but her symptoms lingered for weeks—and then began worsening. Adinig lost weight, developed new severe allergies, had unexplained episodes of paralysis, and became wheelchair-bound. At the same time, she felt her cognitive skills slipping away.
“Until this happened to me, I had no idea that a virus could cause a chronic illness like this.”
–CYNTHIA ADINIG, LONG COVID PATIENT
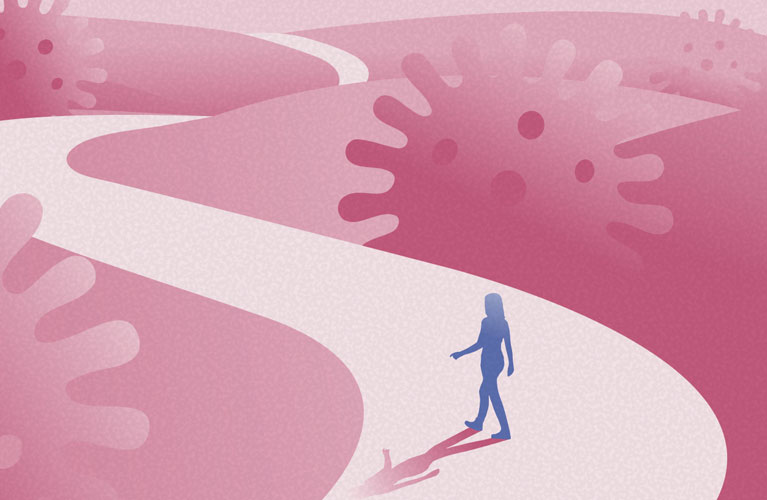
“I used to be the kind of person who memorized pi to the fiftieth decimal place and could recite lines from a television show I had watched one time,” says Adinig. “Suddenly, I couldn’t remember my own address or social security number. I needed help filling out forms because I so often struggled with understanding what was even being asked.”
Four years later, Adinig has still not returned to a full-time job; she has been diagnosed with long COVID and works a few hours a week on long COVID advocacy and outreach efforts. She says she is using techniques like daily puzzles that she learned when her elderly grandmother was diagnosed with dementia to maintain her memory, but she still struggles with brain fog most days and must carefully plan and limit any mental and physical exertion to avoid severe fatigue.
“I’ve always been really on top of science and medicine and new technologies,” says Adinig. “But until this happened to me, I had no idea that a virus could cause a chronic illness like this.”
Adinig is not alone; an estimated 17 million adults have some form of long COVID, which encompasses a variety of symptoms that can last months or years after the initial infection. Clinicians still don’t know who is most likely to develop lasting cognitive symptoms or why. These questions are part of a larger scientific mystery that is just beginning to be tackled: how do the immune system and the brain interact with each other?
“The scientific community is just starting to realize that there are a lot more connections between the nervous system and the immune system than we have ever appreciated,” says Professor Susan Kaech, director of the NOMIS Center for Immunobiology and Microbial Pathogenesis and holder of the NOMIS Chair at Salk. “When our immune system responds to an infection, our brain and cognition are clearly impacted in ways that we don’t fully understand.”
Armed with a new $20 million, five-year gift from the NOMIS Foundation, Kaech and her faculty colleague Associate Professor Nicola Allen are co-leading a new Neuroimmunology Initiative at Salk to focus on this topic. The Initiative will bring together Salk neuroscientists and immunologists, including Professor Axel Nimmerjahn and Associate Professor Diana Hargreaves, to connect the dots between the brain and the immune system.
This research will reveal fundamental insights into human biology that have been historically overlooked at traditional research institutions, where faculty from different disciplines rarely get the chance to interact. By choosing to support such collaborations, Salk is taking a front-row seat in shaping the future of this emerging field.
“Our initial goal is to understand the very basics of how our nervous system listens to our immune system, and vice versa, how our nervous system controls our immune system,” Kaech explains. Their discoveries could guide our understanding and treatment of conditions as diverse as aging, Alzheimer’s disease, Parkinson’s disease, long COVID, cancer, and autoimmune disease.
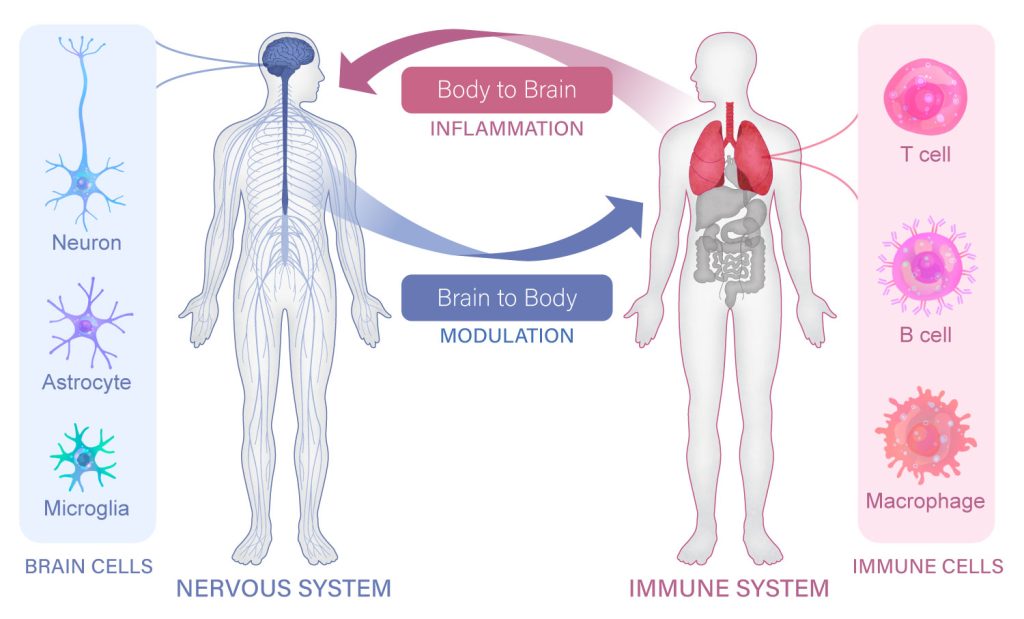
Rethinking the blood-brain barrier
For most of the 20th century, scientists thought the brain and spinal cord were mostly cut off from the body’s immune system, their essential functions protected by a nearly impenetrable wall called the blood-brain barrier. This mindset led to the view that the peripheral immune system likely played a very minimal role in the central nervous system.
“Today, that view is crumbling,” says Nimmerjahn. “We realize there are all sorts of ways that these two systems can communicate, some of which don’t even require immune cells to cross the blood-brain barrier.”
It turns out that the blood-brain barrier acts more like a filter than a wall. Researchers have discovered that immune cells do, during many infections, interact directly with regions of the brain and spinal cord. But they have also realized that the immune system and brain can shape each other in more subtle or indirect ways. There is a constant two-way stream of communication between the two systems, facilitated by intermediary cells and a shared language of signaling molecules.
What do these newly appreciated connections mean for disease and aging? Their very existence could help explain, at a fundamental level, how it is possible for COVID-19 to cause brain fog, or for viral infections to contribute to multiple sclerosis—a link that was only discovered in recent years. They suggest that the immune system may play a role in Alzheimer’s and Parkinson’s diseases and that the brain could contribute to autoimmune diseases and chronic inflammation.
Mostly, the new understanding of the nervous system and the immune system as a tangled, interconnected web of cells and signaling molecules means that scientists need to study the systems together.
“In the past, it’s been a very siloed approach,” says Nimmerjahn. “Neuroscientists have studied cells and cell communication within the brain, but their work ends at the blood-brain barrier. Immunologists have studied what happens amongst immune cells, but again, their focus usually ends at the other side of the blood-brain barrier. We want to bridge that gap.”
Salk researchers are studying how repeated infections impact the brain over time, focusing on memory immune cells that persist in the brain and may affect long-term brain function.
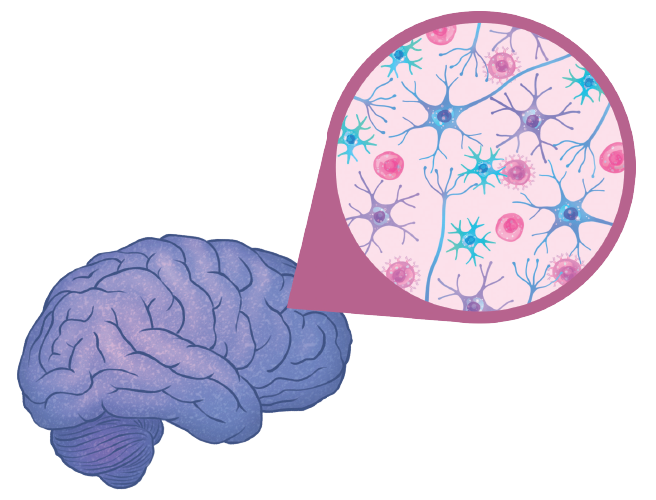
Brain changes
If you’ve ever felt tired, sluggish, forgetful, or unfocused while battling a case of influenza or a common cold, then you already know that a virus can impact your brain and cognition. The first goal of the new Neuroimmunology Initiative is to understand how an infection, or a cumulative history of viral and bacterial infections, can change your brain and spinal cord at a molecular or cellular level.
One reason to suspect that such changes exist is that during an infection, a few types of immune cells can, indeed, cross the blood-brain barrier. But even immune cells that don’t make their way into the brain could have an impact. Scientists have recently discovered that immune cells circulating around the body can interact with nerve endings that extend from the spinal cord out into our organs and extremities. These immune cells can alter the electrical signals that these nerves carry back to the brain, likely sending the message, “We’re fighting something out here,” so that the brain can launch an appropriate response. This might include elevating the body’s heart rate to increase the flow of blood and oxygen, or triggering fatigue to promote rest and recovery.
“If you have an ongoing infection in your lungs, there will be molecules produced by the immune system that circulate all through your body to coordinate the immune response,” explains Kaech. “There’s no question now that those molecules are going to be sensed by the nervous system.”
So when a message makes its way to the brain—either through the long arms of a peripheral neuron or by an immune cell crossing into the brain—what happens?
The Neuroimmunology Initiative team is creating a brain map that they’ve dubbed the “InflamMind” to capture how inflammation throughout the body affects the brain. Nimmerjahn, over the course of his career, has developed microscopy techniques to observe brain and spinal cord cells in living animals. He’ll use those approaches to study how different types of brain cells change physically and functionally in response to viruses or bacteria.
Meanwhile, Hargreaves will use cutting-edge genomics technologies to follow how the cells change their gene activity. She wants to know which genes turn “on” or “off” in immune and brain cells during or after an infection.
“Looking at the gene expression of these cells can give us a clue about how they are responding to infection,” says Hargreaves.
Scientists know that inflammation can remodel the physical structure of a cell’s DNA so that certain genes become more accessible or less accessible. This affects which genes can be expressed and ultimately shapes the cell’s health and behavior. Advanced tools at Salk let Hargreaves and her colleagues observe when a stretch of DNA has loosened to allow certain genes to be expressed, or identify which genes are actively being expressed at any given time. Analyzing the DNA’s structure this way can suggest how sensitive neurons or immune cells in the brain might be and, therefore, how likely they are to launch an immune reaction.
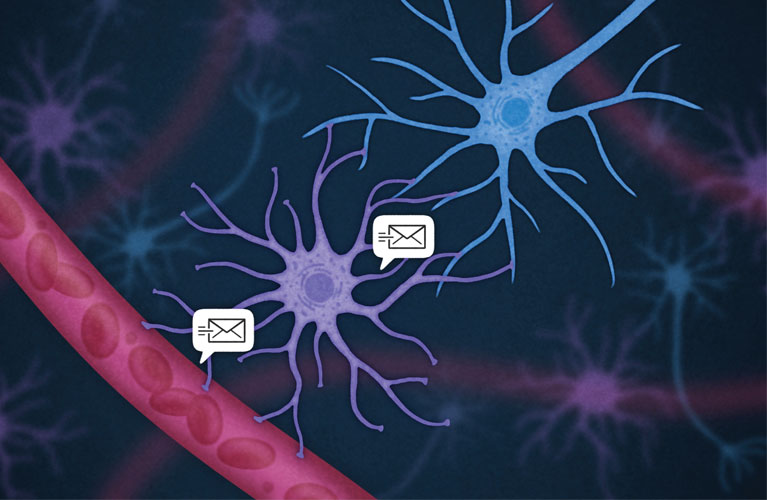
Immune cells (red) send molecular messages to astrocyctes (purple), which can translate them to neurons (blue).
Infection after infection
When Adinig was infected with SARS-CoV-2, the virus that caused her long COVID, it was not the first time she had ever gotten sick in her life. That pattern of repeated infection, while common in people, is rarely modeled in the lab. Salk researchers want to change that.
“Typically, in lab mice, we study the consequences of infection by infecting an animal with one pathogen for a few days, and we leave it at that,” says Hargreaves. “But we, as humans, are exposed to a variety of pathogens throughout our lives, one after the other. What does that ultimately do?”
Studying the effects of a single pathogen is already costly work, so looking at several at the same time presents additional logistical and funding challenges. Such obstacles have precluded these kinds of experiments in the past, but with the NOMIS Foundation’s support, Salk scientists are now accepting the challenge.
By producing more realistic models of pathogen exposure, the Neuroimmunology Initiative will finally be able to look at the cumulative consequences of repeat infections on the brain and spinal cord.
“How is the collective history of multiple infections over our lifetime impacting our central nervous system?” Kaech asks. “There has been nothing done in the past to explore this.”
Kaech has long studied how the immune system develops long-term “memories” of the pathogens it encounters. She says that each time a mouse gets an infection, new immune cells enter the brain—a process her lab staff can observe under a microscope. Moreover, the immune cells persist in the brain even after the animal has recovered, ready to spot the pathogen should it ever be re-encountered.
“These cells are there to protect you if you get that infection again, but we don’t know their long-term consequences,” Kaech says.
Her lab is now working to better understand the activity of these memory immune cells and how they might affect brain function. The work requires time-consuming, expensive experiments that integrate classic immunology with detailed characterizations of animals’ brain cells—the kinds of studies that are not possible without intentionally dedicated funding and support.
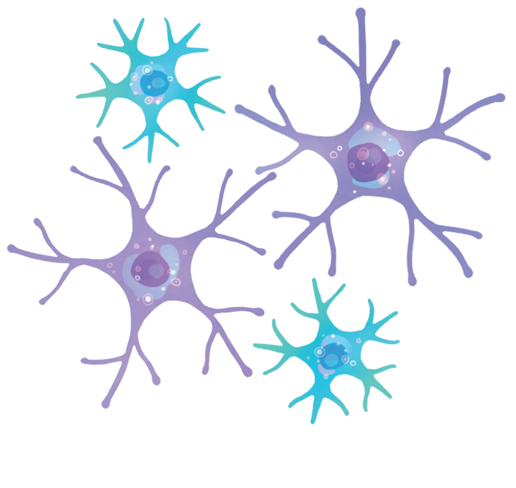
“When we’re talking about communication between two systems, there need to be some cells that can speak both languages. Microglia and astrocytes are those cells in the brain.”
–professor Axel Nimmerjahn
The brain cells to blame
Once Salk researchers identify the brain changes that follow an infection, their next task will be to figure out exactly how they come about. The brain and immune system likely communicate through cells that can transform the immune system’s chemical signals into the nervous system’s electrical messages, and vice versa. Today, scientists know about two types of cells within the brain that can fulfill these roles as translators: microglia and astrocytes.
“When we’re talking about communication between two systems, there need to be some cells that can speak both languages,” says Nimmerjahn. “Microglia and astrocytes are those cells in the brain.”
Allen, the Initiative’s co-director, is an expert on these non-neuronal brain cells. In 2018, her lab discovered that as animals age, their astrocytes change—genes that are turned “off” for most of an animal’s life become reactivated over time. Many of those genes are related to astrocytes’ ability to prune the connections between neurons—an important part of refining neural circuits during early brain development. Allen and her colleagues hypothesize that older astrocytes start to eliminate these connections at higher rates, potentially contributing to cognitive declines.
It is also known that aging is associated with higher levels of inflammation across the body. Because astrocytes also speak the language of immune cells, Allen now wonders whether their changes during aging are a response to these increased levels of inflammation.
“What my lab is following up on, as part of the new Initiative, is whether inflammation is contributing to these changes in older astrocytes,” says Allen. “Are immune cells outside of the brain starting this cascade?”
If she can explain how age-related inflammation alters astrocytes, she may also be able to determine how astrocytes respond to other sources of inflammation, such as a viral infection or a chronic disease. This could inform how viruses like SARS-CoV-2 can trigger cognitive decline in some people or how neurodegenerative disorders could be impacted by immune changes. The key to understanding these diseases, Allen hypothesizes, is to study how astrocytes interact with immune cells and then alter the brain cells nearby.
“In some of these neurodegenerative disorders like Alzheimer’s, we’ve been very focused on the neurons but have ignored the broader picture in terms of what other cells surround these brain cells and interact with them,” Allen says.
Nimmerjahn is tackling the other brain immune cell of interest: microglia. Using his cutting-edge microscopy tools, he has already shown that microglia in the healthy brain are constantly changing shape, stretching their arms in all directions to patrol for threats. He has also examined the cells’ role in diseases, including viral infections and Alzheimer’s disease. Now, Nimmerjahn wants to know how these immune sentinels change with chronic inflammation, and how that could impact such diseases.
Nimmerjahn has also partnered with Salk Professor Rusty Gage, whose lab has pioneered methods for producing tiny clusters of cells that mimic the human brain so that it can be easily studied in the lab. Together, they recently showed that these “brain organoids” can be used to study not only neurons but also astrocytes and microglia. The researchers discovered that, in an organoid grown out of cells from a patient with autism, spectrum disorder microglia were larger and more reactive than usual—suggesting a possibility that the immune system and inflammation may play a role in autism. They plan to repeat similar experiments to study how microglia may function differently in other diseases or inflammatory conditions.
Treating asthma by targeting the brain rather than the immune system or lungs is exactly the kind of new idea that can come from studying these systems more holistically.
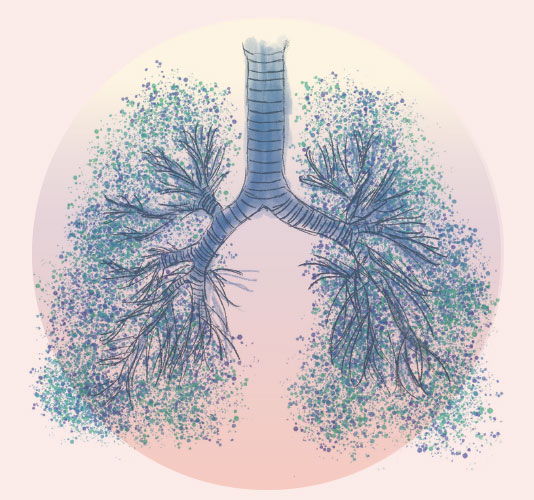
Listening to the brain
After probing how the immune system communicates to the nervous system and how cells in the brain respond, the final aim of Salk’s Neuroimmunology Initiative is to better understand how the brain goes on to control immunity, closing the loop of neuroimmune interaction.
When Kaech opened her lab at Salk, she launched a project to better understand how nerves and immune cells, like T cells, talk to each other. T cells are a type of white blood cell that play a central role in fighting infection and disease. As Kaech tracked the behavior of T cells in various organs during chronic viral infection or cancer, she discovered something surprising: the T cells listened to neurotransmitters produced by nerves located within the organ tissue. Kaech went on to show that the T cells have receptors for these brain signals, letting them eavesdrop on the central nervous system.
“It was amazing to see that these immune cells actually have receptors for neurotransmitters that are produced by nerve cells,” says Kaech. “Not only are the immune system and nervous system not separate, but they have this shared chemical language. It was a pretty big insight.”
Kaech’s group wondered how the T cells would behave if they didn’t receive these messages from the nervous system. To test this, they blocked the cells’ receptors using beta-blockers—a class of drugs commonly used to control blood pressure and heart rate. Surprisingly, the immune cells became better at destroying cancer cells when they no longer received the nerves’ signals. The results were published in 2023 in Nature.
Interestingly, the molecule used as a messenger in this case was noradrenaline, a neurotransmitter typically associated with the body’s “fight-or-flight” response. The research suggests that a person’s stress levels could impact their immune response—something relatable to anyone who has become sicker than usual during exam week or another high-stress time.
Nimmerjahn has also helped scientists learn how our brains can contribute to asthma, a chronic inflammatory disease of the lungs. In this research, led by UC San Diego collaborator Professor Xin Sun, the team studied mice that had been exposed to dust mites, which can trigger asthma in humans. They discovered that when mice repeatedly breathe in these dust mites, immune cells activate nerve cells near the lungs, which then change the activity of neurons in the brain. In turn, those brain cells send signals back to the lungs to trigger inflammation. Over time, the animals’ immune systems became even more sensitive and hyperreactive to the dust mites, eventually resulting in signs of chronic asthma. It was the first time asthma could be linked to a full circuit of communication from the immune system to the brain and back to the immune system in the lungs.
“Once you identify a circuit like that, you can start to think about treatments that potentially regulate it,” says Nimmerjahn.
When the team blocked the communication between brain cells in this circuit, the mice no longer developed asthma in response to the dust mites. Treating asthma by targeting the brain rather than the immune system or lungs is exactly the kind of new idea that can come from studying these systems more holistically.
“We’ve studied both these systems in isolation for a long time. But it’s time to bring the fields together. It’s a big shift in perspective, but that’s often how the greatest innovations begin.”
–Associate Professor Nicola Allen
Breaking the cycle
If drugs targeting the nervous system can prevent asthma, or push the immune system to be better at fighting cancer, what else is possible? Salk scientists say they’ve only scratched the surface of the many conditions that could be treated or prevented by interrupting the crosstalk between our immune cells and neurons.
Recent studies are starting to suggest that people who have had certain viral and bacterial illnesses have higher rates of Alzheimer’s and Parkinson’s diseases later in their lives. Could immunotherapies one day treat or prevent these brain diseases? Could similar drugs stop the lasting brain fog and cognitive dysfunction of long COVID or chronic fatigue syndrome? Could our brains hold the key to treating allergies and autoimmune diseases?
By bringing neuroscientists and immunologists together, the Salk Neuroimmunology Initiative hopes to answer questions like these. Ideas that started as curious conversations in the hallways of Salk have now moved to regularly scheduled meetings, where scientists at the intersection of these disciplines can interact and collaborate. As they learn to speak each other’s scientific languages, they’ll come closer to deciphering the shared language of the brain and immune system.
Featured Stories
Connecting the dots—From the immune system to the brain and back againBy collaborating across disciplines like genetics, neuroscience, and immunology, Salk scientists are uniquely positioned to lead us into a future of healthier aging and effective therapeutics for Alzheimer’s.
Salk mourns the loss of Joanne ChorySalk Professor Joanne Chory, one of the world’s preeminent plant biologists who led the charge to mitigate climate change with plant-based solutions, died on November 12, 2024, at the age of 69 due to complications from Parkinson’s disease.
Talmo Pereira—From video game bots to leading-edge AI toolsTalmo Pereira is a Salk Fellow, a unique role that empowers scientists to move straight from graduate school to leading their own research groups without postdoctoral training.
Kay Watt—From Peace Corps to plant scienceAt the heart of the Harnessing Plants Initiative is Program Manager Kay Watt who tackles all of the strategy, site operations, budgeting, reporting, communication, and outreach that keep the whole program on track.
Pau Esparza-Moltó—Seeing mitochondria as more than just a powerhousePau Esparza-Moltó, a postdoctoral researcher in Professor Gerald Shadel’s lab, finds comfort in the similarities between his hometown in Spain and San Diego, where he now studies cell-powering mitochondria.
Salk summer programs bring equity and opportunity to the STEM career pipelineThe Salk Institute recently hosted two inaugural events designed to enhance diversity within the scientific community: the Rising Stars Symposium and the Diverse Inclusive Scientific Community Offering a Vision for an Ecosystem Reimagined (DISCOVER) Symposium.