FRONTIERS Harnessing plants for the future
Get ready, sunny San Diego: Winter is coming.
We’re not talking about a new season of Game of Thrones—although the story is one of epic proportions and high stakes.
Salk Institute scientists will soon have the ability to control the weather, to create at will frigid northern winters, baking desert summers and steamy jungle monsoons. In fact, they will be able to simulate nearly any climate on Earth in a state-of-the-art plant research facility slated to begin operation in November 2017.
In the facility’s high-tech grow rooms and chambers, Salk’s plant biologists will put plants through intense paces, subjecting them to temperature, light and moisture conditions that mimic everything from Spanish summers to Swiss winters. The new facility anchors the Institute’s Harnessing Plants Initiative, an ambitious effort launched this fall to develop biology-based solutions to the urgent challenge of global climate change, one of the most serious threats facing humanity.
“Our world is at a crossroads… Our action or inaction will determine our fate.”
Joanne Chory – Professor and Director, Plant Molecular and Cellular Biology Laboratory
“Our world is at a crossroads,” says Joanne Chory, director of Salk’s Plant Molecular and Cellular Biology Laboratory, Howard Hughes Medical Institute (HHMI) investigator and mastermind of the initiative. “Over the next fifty years, the human population will grow to around 10 billion people. Right now we have 7 billion people and we have already strained the planet’s ability to support us. Our action or inaction will determine our fate.”
Chory, who holds the Howard H. and Maryam R. Newman Chair in Plant Biology, and her colleagues have chosen action. Over the past decades, Salk’s plant biology team has emerged as a world leader in pioneering discoveries about the inner workings of plants. They have illuminated the molecular machinery inside plant cells that allows them to grow, survive stress and adapt to new environments. Now, Chory and her colleagues plan to apply their hard-won foundational knowledge of plant biology to the practical purpose of addressing the myriad disruptions posed by global warming.
The Harnessing Plants Initiative hinges on developing “ideal plants” to help tackle the critical and interlinked challenges of human emissions of carbon dioxide, declining agricultural yields and collapsing ecosystems. At the same time, these ideal plants will help meet the burgeoning demands of a rapidly growing human population for plant products.
Salk has a two-pronged solution:
- Use “ideal” terrestrial crop plants to capture a significant portion of human-emitted CO2.
- Maintain sequestration capacity of coastal marine environments by reversing seagrass loss.
SOLUTION 1:
Breaking the cycle
Utilizing suberin to store more carbon without releasing it
Carbon Conundrum
Life on Earth begins and ends with carbon. The stuff of life—proteins, carbohydrates, lipids and DNA—is built on a carbon backbone. Life emerged around three billion years ago and has since evolved into billions of species. The vast majority of species that have existed have since gone extinct. Interred in the earth ages ago, certain forms of life—plants and algae, in particular—left behind enormous concentrations of carbon: coal, petroleum and natural gas. Carbon made life possible, and the end of uncounted lives made concentrated, energy-rich carbon abundantly available for the most enterprising of species, Homo sapiens.
Since the dawn of the Industrial Revolution, humans have become very good at exhuming the remains of our carbonized predecessors for use as energy to power our modern societies. Too good, it seems. Over the past century, the concentration of greenhouse gases in the atmosphere has steadily increased, mostly from burning fossil fuels. Currently, humans emit around 35 gigatons of carbon dioxide into the atmosphere annually through industry, transportation, electricity generation and other activities. Greenhouse gases prevent light from the sun from reflecting back into space, effectively trapping heat. The result has been a 1.5-degree increase in average global surface temperature since the late 19th century—global warming.
The impact of this warming is unmistakable. The polar ice caps are melting with alarming rapidity and the oceans are swelling as they warm. Both factors raise sea level, which threatens coastal communities with erosion and flooding, and the warming oceans fuel massive storms and extreme weather. Recent summers have been the hottest and driest on record, resulting in increasing numbers of forest fires.
The same conditions are suppressing global agricultural yields, a trend that is likely to worsen if predictions of an additional global warming of 2 to 3 degrees over the next few decades prove accurate. “The human population will grow by around 40 percent over the next 50 years and the middle class may double,” says Chory. “This puts human consumption and global warming on a dangerous collision course.”
Many efforts are under way to reduce the amount of carbon we emit, but these initiatives have run into political, economic and technological obstacles. It has become clear, says Chory, that solely focusing on reducing emissions won’t solve the problem. We simultaneously need to focus on removing the carbon that has accumulated in the atmosphere. By developing specialized crops capable of adapting to a changing climate, Chory and her colleagues believe they can substantially increase the amount of carbon captured and stored in the planet’s soils. At the same time, they plan to identify varieties of seagrasses to maintain and restore coastal aquatic ecosystems, where massive amounts of carbon are stored in the roots of these grasses.
Corking Carbon
Plants are excellent at scrubbing carbon from the atmosphere. Every year, the world’s plants breathe in around 64 gigatons of CO2 from the air, storing the carbon in leaves, shoots and roots. To get a sense of the staggering amount of carbon plants absorb, consider that an adult African elephant at the San Diego Zoo can weigh up to 6.8 metric tons. One gigaton is equivalent to more than 100 million of those elephants. Now multiply by 64.
While plants store this carbon in the form of numerous biomolecules, almost all of these materials are degraded by animals, fungi and bacteria and the stored CO2 thereby released. The Salk team has identified one particular plant-made molecule, called suberin, that is highly resistant to this degradation and can thereby remain in the soil. Suberin, better known to wine aficionados as cork, is a waxy, water-repellant and carbon-rich substance, and is at the heart of the Salk team’s strategy to address the problem of meeting human needs while reducing carbon in the atmosphere. Each growing season, annual crops—plants that live only one season, such as corn or wheat—breathe in CO2 to build plump leaves, tall stems and thick roots.
At the end of the season, the plants die and rot, releasing much of that carbon back into the atmosphere.
“What if you could tweak this carbon cycle in the plant so that it absorbed more carbon and released less?” says Joseph Noel, a member of Salk’s plant biology team and Howard Hughes Medical Institute investigator.
“We realized that a crop with a larger, suberin-dense root would capture more carbon in the ground,” he says. “Roots last longer than other parts of plants, particularly in perennial plants that live multiple years. Even in dead roots, suberin decays very slowly.”
Carbon stored in suberin could potentially stay in the soil for hundreds or even thousands of years. So you have two possible benefits: store more carbon and store it for a long time. An expert on the structure and chemistry of compounds produced by plants and on how plants have evolved unique ways to make their own specialized products to adapt to nearly every ecosystem on Earth, Noel is exploring how plant cells can be coaxed to produce more suberin—and thus capture more carbon.
Increasing the amount of suberin each cell produces is one strategy; another is to increase the number of cells—in other words, to grow larger roots. In both cases, the newest addition to Salk’s plant biology team, Associate Professor Wolfgang Busch, is providing critical expertise. Busch combines techniques from genetics, genomics and other science fields to understand how root growth in given environments is determined by a plant’s genes.
“There is still a great deal we don’t know about how genes and molecular mechanisms determine how a plant root decides to grow to a certain size or in a certain direction in the soil,” Busch says. “To breed crops with large roots that produce a lot of suberin, we’ll need a better understanding of how to influence both aspects at the genetic and molecular level.”
In addition to his expertise, Busch brought a plant with him to Salk: Lotus japonicus. A native plant of Japan, known commonly in English as birdsfoot trefoil, Lotus is a wild legume that has become the laboratory stand-in—a “model organism” in scientific parlance—for studying the biology of all legumes, a group of plants that includes chickpeas, beans, peanuts and lentils. In their quest to develop the ideal plant, the Salk team will experiment with both Lotus and Arabidopsis thaliana, the common mustard weed that is the predominant plant research model. Much of what scientists know about the fundamentals of plant molecular and cellular biology has come from studying Arabidopsis. Adding Lotus to the research mix will allow the Salk team to expand its research to focus on a plant more closely related to crops. This is important to the Harnessing Plants Initiative, as the carbon-capturing plants the Salk team is designing would need to be food-producing crops deployed at a scale sufficient to put a significant dent in global warming.
“Most of the world’s arable land is already being used for agriculture, and we are already straining our natural ecosystems and our capacity to generate food and other plant products,” says Busch. “A legume crop bred with a large root could be planted on farms around the world and could potentially have multiple advantages over current crops.”
Deploying an “ideal” legume crop on a relatively small portion of the world’s farmland could capture a significant portion of global CO2 emissions. Among the other advantages of legumes is that crops could be perennials, surviving and producing year after year, which avoids annual replanting. The roots would remain undisturbed, keeping the plant’s carbon haul locked underground. Legumes also tend to make friends. Most grow nodules on their roots as homes for bacteria called rhizobia, which convert nitrogen from the air into usable forms the plants need to thrive. This is why farmers include legumes in crop rotations with other plants to help replenish soil nitrogen. The crops envisioned by the Salk team thus require less fertilizer than normal crops, reducing the harmful farm run-off that damages aquatic ecosystems.
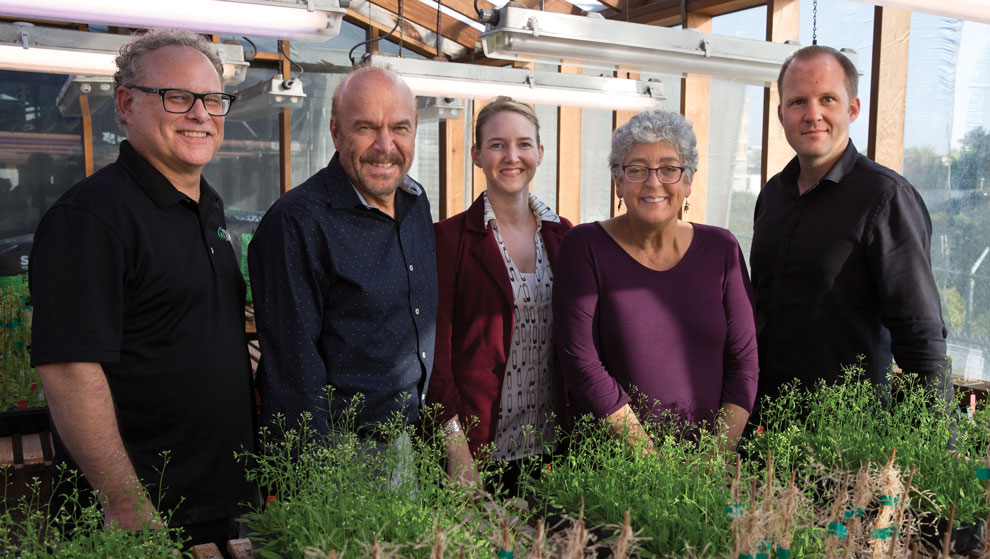
Learning from Nature
Salk’s new plant growth facility will be critical to finding ways to turn crops into carbon warehouses. Just as important, it will help the researchers find ways to grow plants in a variety of conditions. Plants are highly attuned to specific climates, and subtle variations in temperature, rainfall, humidity and soil salinity can have a serious impact on their growth. Even within a species, plants can prefer very different environments. Arabidopsis, for instance, grows naturally around the globe, in climates ranging from arid regions of northern Africa to temperate northern Europe. In Japan, Lotus grows from tropical Okinawa to frigid Hokkaido, the northernmost of the country’s major islands.
Julie Law, another member of the Salk plant biology team, says the natural variation that allows plants to live in very different conditions is a kind of evolutionary playbook that the Harnessing Plants Initiative can draw from. “As plants spread and adapted to new conditions, they evolved new survival skills,” says Law, an assistant professor at Salk. “We can learn from these plants to design crops that will be highly adaptable and can grow in a range of climates.”
For instance, the climate of the Midwest, the traditional breadbasket of the United States, is becoming hotter and drier. At the same time, flooding due to heavier rains has increased. Looking globally, a 2016 study estimated that drought and extreme heat reduced worldwide crop yields by as much as 10 percent between 1964 and 2007. Many plants developed to capture and store large amounts of carbon will need to be heat and drought tolerant to thrive. Resilience to flooding might also come in handy, as extreme rain events and resulting floods are also a growing threat to crops. By collecting varieties of Arabidopsis and Lotus from a range of different climates, then growing them under hot, dry conditions in the new climate simulation facility at Salk, the Institute’s plant biologists can identify the plants best adapted to that situation. They can look for what makes plants resistant to flooding or cold snaps—or any other climate threats that seem to be increasing in frequency. They can then analyze the genetic and molecular makeup of these plants to find out what makes them durable, and use what they find to breed those traits into their “ideal plants.”
“The ideal plant doesn’t just capture carbon and produce food,” says Law. “It also grows where you want it to grow. As climate changes, many regions are becoming hotter and drier, but others are getting colder and wetter. We need a toolbox of adaptive traits to draw from.”
In addition to identifying key genetic traits for developing ideal plants, Law and Joseph Ecker, another Salk professor and HHMI investigator, are searching for key patterns of epigenomic modifications, chemical changes that alter a plant’s genetic activity without changing the letters of the DNA alphabet (A-T-C-G). These patterns have allowed plants in nature to adapt to various environments, and will likely be crucial to developing the ideal plants envisioned by the Salk team.
“The essence of climate change from a plant’s perspective is responding to stress, because changes in the plant’s environment can represent an existential threat,” says Ecker. “Developing a thorough understanding of the genetic and epigenetic mechanisms that control plants’ stress responses will allow us to develop crops that are more resilient and precisely suited to specific climate regimes.”
SOLUTION 2:
An unexpected ally
Costal marine plants can be transformative.
An Aquatic Ally
In addition to land plants, the Salk team plans to extend their research to seagrasses, one of the other major repositories of the planet’s carbon. Coastal seagrass beds store nearly twice as much carbon per acre as terrestrial forests and account for about 10 percent of the carbon stored in the ocean. Unfortunately, due to dredging and pollution, seagrass ecosystems are seriously threatened around the world. About 1.5 percent of these ecosystems disappear each year. Changing water temperatures due to global warming accelerates this decline. As they go, so goes a range of important functions they serve: carbon sequestration, habitat for fish, and storm and flood protections for coastal communities.
Maintaining existing seagrass ecosystems and restoring others, says Noel, offers a clear-cut solution to addressing climate change. Similar to land plants, seagrasses grow in a range of climates, so as water conditions change and kill off grasses in a region, scientists can draw from the natural variation to identify replacements from other areas that are more likely to survive the new normal. A tropical seagrass, for instance, might grow well in an area where temperate waters are warming. Another tactic might be to restore ecosystems with carbon-hungry varieties of seagrasses.
“Certain varieties of seagrass have greater carbon storage capacity,” adds Noel. “If this trait was bred into other varieties of grasses, we could sequester far more carbon in coastal ecosystems.”
The Salk team plans to identify what traits make certain seagrasses suited to various ecosystems, and to use that knowledge to identify what varieties could be transplanted to ecosystems where climate change is killing grasses. Restoring compromised aquatic ecosystems would have the double benefit of capturing more carbon in the sea and reestablishing ecosystems, such as coral reefs, that support fisheries.”
“That’s the beauty of both our terrestrial and our aquatic strategies,” says Noel. “They address the food problem and the climate change problem simultaneously, on the land and in the water. We humans are going to need to be very smart to get through the next century, and that means developing strategic solutions based on good science.”
Support a legacy where cures begin.
Featured Stories
Harnessing plants for the futureGet ready, sunny San Diego: Winter is coming. We’re not talking about a new season of Game of Thrones—although the story is one of epic proportions and high stakes.
Sung Han was destined for SalkHan, an assistant professor in the Clayton Foundation Laboratories for Peptide Biology, arrived at the Institute a year ago to study how the brain recognizes aversive sensory signals.
All roads lead to science for Elena Blanco-SuárezFrom early on, it was pretty much a given that Elena Blanco-Suárez would be a scientist.