Frontiers The weed that changed the world
How Arabidopsis thaliana became one of the most important tools in science
Around Easter in 1905, a 20-year-old German botany student named Friedrich Laibach picked a small, flowering weed from the bank of the Lahn River near his hometown of Limburg, placing it in a solution of acetic acid for preservation.
Back in the lab at the University of Bonn, Laibach stained the plant’s cells with dye and examined them under a microscope, noting that they had only five chromosomes. This excited his advisor, as it was the lowest odd number of chromosomes known to science at the time. (A plant with four chromosomes had been identified the year before.) The plant was Arabidopsis thaliana and—although the exact spot where Laibach collected it was paved over for the Autobahn in the 1930s and lost to history—the species has since become firmly rooted in the pantheon of biology’s most important research tools.
Today, Laibach is credited with making Arabidopsis thaliana, a nondescript member of the mustard family, a model organism: one used to study basic biological functions, which may be applicable more widely. It’s a mainstay of plant biology and genetics research owing not only to its small chromosome number but also to its compact size, rapid life cycle and prolific seed generation. In other words, it’s relatively fast to sequence the plant’s genome, it doesn’t take up much lab space and multiple generations can be grown quickly, saving time and resources.
In 2000, Arabidopsis thaliana was one of the first organisms—and the first plant—to have its genome sequenced, beating the fruit fly, mouse and human to the punch. The seven-year, $70 million Arabidopsis Genome Initiative (AGI) involved multiple labs in Europe, Japan and the US working together to determine the DNA sequence of all five Arabidopsis chromosomes—the exact string of chemical building blocks (known as As, Ts, Cs and Gs) that spell out the plant’s genetic code.
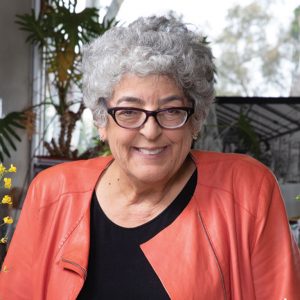
Joanne Chory
Salk Professors Joanne Chory and Joseph Ecker were instrumental in getting the initiative off the ground in the US, rallying influential Arabidopsis researchers around the idea, which led to significant funding with the National Science Foundation as the lead agency. (At the time, Ecker was at the University of Pennsylvania; he arrived at Salk in 2000.)
“In the early ’90s, it was pretty clear that all of the model organisms at that time—yeast, fruit fly, worm and mouse—were headed toward having their genomes sequenced because it was really challenging to do science otherwise,” says Ecker, who directs Salk’s Genomic Analysis Laboratory, holds the Salk International Council Chair in Genetics and is a Howard Hughes Medical Institute investigator.
“So, those of us in the Arabidopsis community were asking, ‘Are we going to let those other groups beat us to sequencing?’ We wondered if people would even want to work on plants if there were better tools for other model systems.”
After the initiative got underway, Ecker co-led a team of US-based researchers to sequence chromosome 1, the largest of the five Arabidopsis chromosomes. The other four chromosomes were sequenced by teams from the US, Japan and the European Union.
Chory, who made a big impact in plant biology with a 1989 paper revealing an Arabidopsis mutant that could grow in the dark, was asked to be a member of AGI’s scientific advisory committee. “When I first arrived at Salk, we published a paper on a mutant called DET1. This mutant could grow in the dark like a light-grown plant, even though it was not exposed to any light. What was unique about our study was the application of genetic techniques to a problem that had been studied only by plant physiologists. It was the beginning of a huge revelation of how complex the pathways are that allow a plant to respond to light,” says Chory, director of Salk’s Plant Molecular and Cellular Biology Laboratory, Howard H. and Maryam R. Newman Chair in Plant Biology, and Howard Hughes Medical Institute investigator. “It was really hard to clone [identify] a gene with the tools that we had back then, and no overall sequence.”
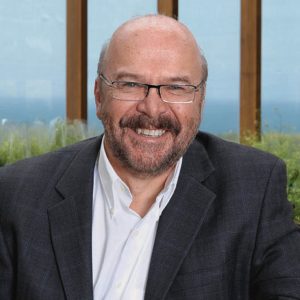
Joseph Ecker
Deciphering the Arabidopsis genome was revolutionary for both plant biology and genetics. Before that, plant biologists who had an interesting mutant had been unable to clone genes to understand the molecular underpinnings (the mechanism) of how a mutation in such a gene could cause a dramatic phenotype—growing in the dark, for example, or tiny leaves. (In genetics, these known mutations are called markers.) The frequency with which the mutations show up together (dark-growing plants with tiny leaves) in the offspring can be used to map the genes relative to each other, although it takes many crosses (breeding plants)—with multiple markers—to get close to the gene of interest. In this way, and with a molecular technique called RFLP analysis, Chory was able to localize the DET1 gene to the top of chromosome 4.
Identifying the gene’s chromosome was only the first step; figuring out the sequence and exact location in the genome was another laborious undertaking, which Chory accomplished for DET1 in 1994. In part, this involved searching a library of DNA fragments for one that overlapped the nearest marker. Then the nonoverlapping end was used to again search the library for another overlapping fragment. Repeated over and over, this process—known as chromosome walking—could eventually reach the gene of interest. Identifying and mapping a single gene within the genome could take a long time.
“I had a headache for six years,” says Chory. “It was so much work.”
The AGI made it possible for researchers to locate genes much more easily within the Arabidopsis genome. They could, for example, look for DNA sequences that are telltale signs of genes. Or they could look for gene sequences already known from other plants and see if they were also present in the Arabidopsis genome.
Most importantly, researchers could use a method called plant transformation to identify unknown genes in the genome. One of the keys to this method is a bacterium called Agrobacterium tumefaciens, which infects plants. The bacterium does so to randomly insert its own DNA, in the form of a circular structure called a plasmid, into the plant’s genome. The bacterial DNA causes the plant to form a crown gall or tumor.
Scientists figured out how to replace the bacterial DNA with custom DNA (known as a T-DNA insertion line), which Agrobacterium ferries into the plant. (Viruses are used in a similar way to deliver gene therapies into human cells.) If the T-DNA lands in a gene, it can cause a mutation—for example, a plant that produces petals where the stamens would normally be. Then scientists can locate their T-DNA within the genome and know that it must have landed in a gene related to flower development. They can sequence that region and identify the gene.
With this approach, using hundreds of thousands of T-DNA insertion lines, scientists have managed to identify, to some degree, all of Arabidopsis’ approximately 28,000 genes.
One of the scientific community’s most important T-DNA insertion collections, or sequence-indexed insertion mutant libraries, was created by Ecker and other Salk researchers. These insertions are known as “Salk lines.” Younger plant scientists and geneticists may not have heard about Jonas Salk and the polio vaccine, says Ecker, but they’re very familiar with Salk lines, which can be ordered from a Salk website. To date, the database has been accessed more than 11 million times, typically 4,000 times per month. Salk also provides seeds to the global Arabidopsis research community via two seed banks.
“If we understand all these unique features of plants—the edge cases of how plants have adapted to very specific environments or specific physiologies or very specific architectures—then we can start to use that knowledge to build plants that do the specific things that we need.”
– Todd Michael
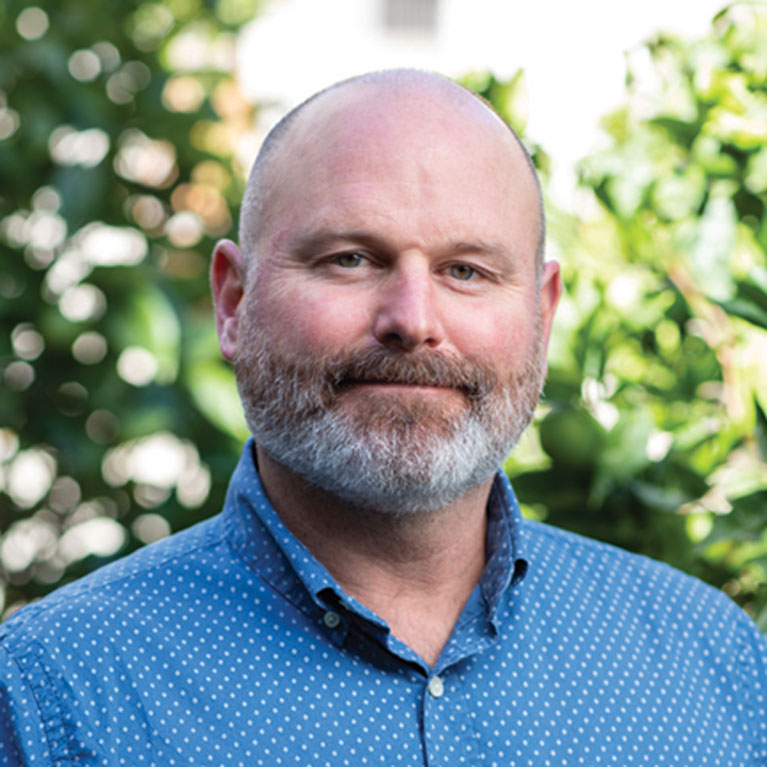
Branching Out
The original AGI didn’t assemble the entire Arabidopsis genome; it left some regions unfinished that were challenging to complete with the methods of the time. These included the centromere, a constricted area near the middle of a chromosome that is important for cell division. Recently, Salk Research Professor Todd Michael collaborated on a project to sequence and assemble the Arabidopsis centromeres, which had been hard to do previously because they contain many long, repetitive segments, and earlier sequencing techniques were better suited to deciphering short, variable segments.
An expert in genome sequencing, assembly and analysis, Michael has branched out from Arabidopsis. In 2015, he published the first near-complete genome of the drought-tolerant grass Oropetium thomaeum, which revealed complete centromere structure for the first time. More recently, his lab sequenced quillworts, small aquatic plants with a unique method of photosynthesis, and Wolffia, the world’s fastest-growing plant, known colloquially as duckweed. He is also working on a collaboration to sequence the pan-genome of sorghum, one of the top five cereal plants in the world. Sorghum is drought-resistant and grows well on marginal land, making it agriculturally important in a warming world. Although sorghum was initially sequenced a decade ago, scientists want to sequence many more breeding lines and wild relatives. The pan-genome comprises the complete set of genes within a species and allows scientists to accelerate the breeding of new disease-resistant, drought-tolerant and higher-yielding varieties.
Michael’s goal in sequencing structurally and functionally unique plants is to discover how genomic differences enable plants to better respond to and exploit their environment. Such knowledge is vital to the Harnessing Plants Initiative, Salk’s approach to mitigating climate change by developing crops and wetland plants that can capture excess carbon dioxide from the atmosphere and store it underground in long-lasting roots.
“The holy grail of agriculture is to design plants that do what we want them to do,” says Michael. “If we understand all these unique features of plants—the edge cases of how plants have adapted to very specific environments or specific physiologies or very specific architectures—then we can start to use that knowledge to build plants that do the specific things that we need.”
Prior to joining Salk, Michael worked for a time at the agricultural biotechnology company Monsanto, where one of his projects was to sequence Arabidopsis strains other than the original strain sequenced in the AGI. Just as no two humans (except for identical twins) have exactly the same DNA, different strains of Arabidopsis also have slightly different DNA. Some strains may be more drought-tolerant than others or more disease-resistant. Some may bloom earlier or have longer roots. Ecker co-initiated a project with European and American colleagues called the 1,001 Genomes Project to sequence the genomes of many different strains and involved Michael in the effort. (The name was a playful nod to the human genome sequencing community, which had a 1,000 Genomes Project underway.)
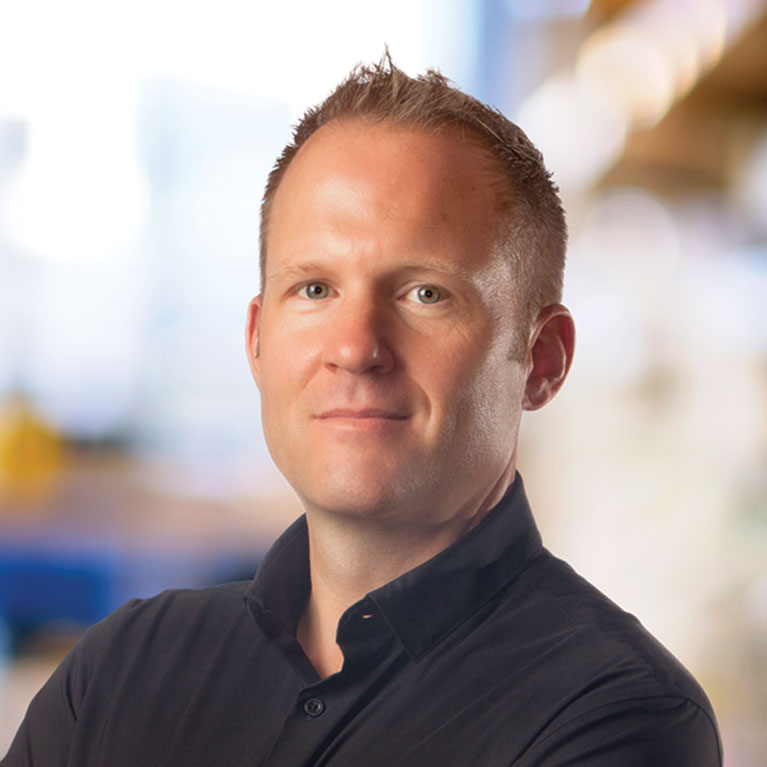
“The hope is to use genes to engineer crops with more and deeper roots that can store more carbon captured from the atmosphere.”
– Wolfgang Busch
Professor Wolfgang Busch, who along with Chory is codirector of Salk’s Harnessing Plants Initiative, is one of the leading experts in using a method called genome-wide association studies (GWAS) to find genes and mechanisms that are responsible for plant features in various strains and has pioneered these approaches for root characteristics. A major component of his success is to use an integrated approach leveraging genetics, genomics and computational approaches along with molecular and cellular biology to learn how root characteristics are encoded in the genetic blueprint of plants.
Over the years, Busch has identified numerous genes using this approach, including a gene that makes roots deeper—a major success for the Harnessing Plants Initiative. The hope is to use genes to engineer crops with more and deeper roots that can store more carbon captured from the atmosphere. He has already taken an important next step in this work, as he is using his arsenal of tools to work on all Harnessing Plant Initiative target crop species. He conducts experiments not only in the laboratory and the greenhouse but also at multiple field locations in the US and South America.
“I started my scientific journey into the systems biology of plant development during my PhD at the Max Planck Institute for Developmental Biology in Tübingen, Germany, in Detlef Weigel’s department,” says Busch, who holds the Hess Chair in Plant Science. “Detlef had just moved there from Salk, so of course I got immersed in all these stories about the exciting plant science that had been going on at Salk. And then, almost all the mutant lines I worked with were from the legendary Salk T-DNA mutant collection that Joe Ecker had established. Salk was all around me already back then.”
Going beyond genes: Epigenetic Post-it notes
Though much of the work of Salk’s plant biologists concerns genetics—DNA sequences and the genes they encode—another fraction involves epigenetics, the various mechanisms that turn genes on or off without affecting the underlying DNA sequence. Epigenetic modifications are how different types of cells—root cells, stem cells, leaf cells—can all have the same DNA but function differently.
Associate Professor Julie Law studies epigenetic modifications using Arabidopsis because it’s such a good model for genetic experiments.
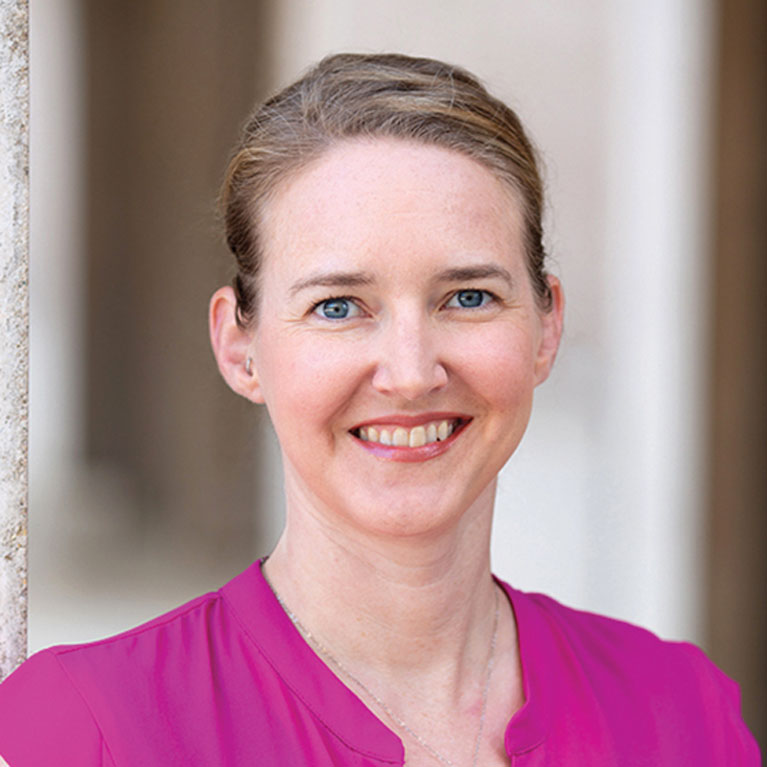
Julie Law
“Mutations that would be lethal in animal cells can be studied effectively in plants,” says Law. “And their short life span allows scientists to study the effects of experimental manipulations over multiple generations and collect a large amount of epigenetic information over a short time.”
Her work has important implications for the Harnessing Plants Initiative, which may be able to use genetic and epigenetic methods to further increase the carbon storage capacity of plants.
Law’s research also has relevance for human health and disease because the same epigenetic processes that are at work in plant cells are also at work in human cells—heart, liver and skin cells all have the same DNA, but they have different functions based on which of their genes are active.
Recently, Law’s lab published a study of how genes in the Arabidopsis CLSY family (pronounced “classy”) control DNA methylation patterns during plant development. DNA methylation is a type of epigenetic modification in which a chemical tag called a methyl group is attached to DNA like a Post-it note reminder that says, “Keep off.” Depending on the plant tissue, different CLSY family members take the lead on targeting where DNA methylation is located, and this results in different patterns of DNA methylation in different plant tissues. Given the roles of DNA methylation in keeping the correct set of genomic regions turned off, these findings may open the door to advances in many areas, from boosting crop yields in plants to informing precision medicine in humans.
Joanne Chory and Joseph Ecker clarified another epigenetic mechanism in a 2021 paper showing that shade from close-growing neighbors causes plants to grow taller. The study looked at the role of proteins called transcription factors in activating this growth response. Transcription factors turn genes on or off by binding to DNA. The team worked with mutant Arabidopsis seedlings that lacked transcription factors called PIFs. When the team grew these plants in simulated shade, the plants without certain PIFs did not elongate or speed up their growth, suggesting that those PIFs are necessary for rapid growth. Further experiments showed that within five minutes of shade onset, the PIF7 protein gets activated and removes an additional epigenetic stop sign called H2A.Z. With the brakes off the growth genes, shaded plants are free to shoot up.
Chory says the work shows how plants respond to subtle environmental changes on the cellular level, as will increasingly occur as plants adapt to global climate changes.
“Wetland plants sequester as much as 100 times more carbon per acre than dry land plants, so an important part of our work in the Harnessing Plants Initiative is to study the genes and genomes of wetlands plants to inform increasingly critical wetland restoration efforts.”
– Joseph Noel
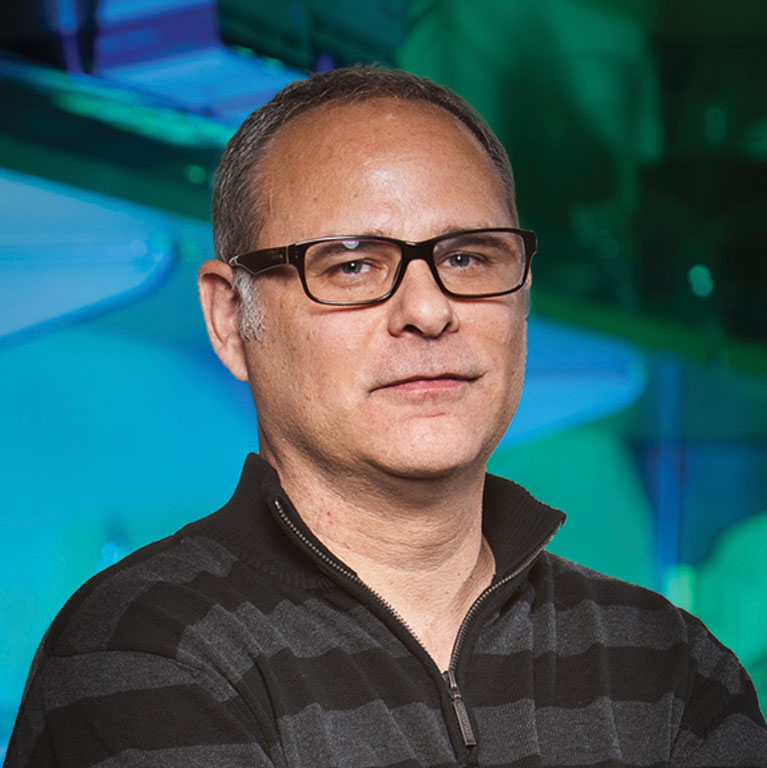
Adapting and advancing
Professor Joseph Noel, also a member of Salk’s plant biology team, is interested in how plants have already adapted to nearly every ecosystem on Earth by evolving unique ways to make their own specialized products. Although he has employed Arabidopsis in the past, he more typically uses biochemistry techniques to study the structure and chemistry of compounds produced by many types of plants.
For example, as part of the Harnessing Plants Initiative, Noel analyzes suberin, the carbon-rich molecule that protects plants from environmental stressors such as drought, floods, disease and salt. It’s found in cork as well as cantaloupe rinds and avocado skins. Noel, director of Salk’s Jack H. Skirball Center for Chemical Biology and Proteomics, is the lead researcher for the Harnessing Plants Initiative’s Coastal Plant Restoration project. This effort aims to develop wetland plants that hold carbon, purify water, preserve land and can thrive in challenging environments around the world.
“People often don’t realize the importance of wetlands in addressing climate change,” says Noel, who holds the Arthur and Julie Woodrow Chair. “Wetland plants sequester as much as 100 times more carbon per acre than dry land plants, so an important part of our work in the Harnessing Plants Initiative is to study the genes and genomes of wetlands plants to inform increasingly critical wetland restoration efforts.”
Noel and Michael routinely don waterproof pants and wade into San Diego’s marshes to collect wetland plants to sequence back in the lab.
Sequencing has advanced by leaps and bounds since 2000, when the first Arabidopsis genome was published. The AGI was an international collaboration that took seven years and $70 million; today, Ecker can sequence an Arabidopsis genome in three minutes for around $16 using a printer-sized machine outside his office. And it will be done more thoroughly that way, he says, because the machine will run through the genome 30 times to ensure high accuracy. “Now that’s a model for success.”
From lab to greenhouse to field
The goal of Salk’s Harnessing Plants Initiative is to use two of Earth’s existing carbon storage mechanisms—plant roots and wetlands—to help mitigate climate change. Salk plant biologists are working hard to optimize crops (Salk Ideal Plants™) to absorb an increasing amount of excess carbon from the atmosphere and store it in the ground, in roots that have increased capacity and can bury the carbon deep.
But to be successful, their research efforts will need to continue to scale up.
In 2016, when Salk plant scientists identified climate change as an important area of research, just four faculty members were studying plants. The labs of Professors Joanne Chory, Joseph Ecker, Joseph Noel and Associate Professor Julie Law had approximately 50 total members. Their labs were in three different buildings (one of which was a trailer), and plants were grown in two scenic but rundown greenhouses perched above the Pacific Ocean at the west end of the campus.
A lot has changed.
Professor Wolfgang Busch and Research Professor Todd Michael joined the plant biology faculty, and together the six labs now have approximately 90 members. Four large (walk-in) and 12 smaller climate-simulation chambers have been constructed in Salk’s East Building, allowing the plant team to reproduce the climate of almost any location on Earth. A custom-built seed-planting robot designed by Busch can accomplish in one day what would take a human researcher five weeks. A state-of-the-art, 10,000-square-foot offsite greenhouse allows researchers to rapidly move discoveries from the laboratory into the greenhouse. Small-scale field trials of Salk Ideal Plants™ have begun in several locations across the US and abroad. And the trailer is being retired.
The rapid growth of the Harnessing Plants Initiative program came when Chory was selected as a beneficiary of the 2019 TED Audacious program, which resulted in the Harnessing Plants Initiative program receiving more than $35 million in new donations. The program has gained additional support since then from individual donors, foundations and businesses, including the Bezos Earth Fund, Sempra Energy and Hess Corporation.
But cooling our warming planet is a monumental challenge. Salk will need to add research personnel, emerging technologies and expanded research space.
Last fall, Salk launched the $500 million Campaign for the Future, a philanthropic and scientific campaign to acquire the people, technology and space necessary to expand Salk’s research to change the world for the better. In addition to recruiting new faculty and leveraging emerging technologies, the Campaign includes the construction of the Joan and Irwin Jacobs Science and Technology Center on the Salk campus. The Jacobs, longtime Salk supporters for whom the Center is named, will donate $1 for every $2 raised from other sources, up to a $100 million. The Institute has until September 30, 2022, to meet the match.
Designed to create research space with the same principles Jonas Salk and architect Louis Kahn applied to the original buildings, the new center will allow the Institute to add scientists from multiple disciplines; expand collaborations between plant scientists, biologists, bioengineers and information theorists; and provide the critical space to work together on the world’s most challenging problems.
Once completed and fully staffed, the Joan and Irwin Jacobs Science and Technology Center will provide a home for the Center for Plant Biology, one of four Salk Centers of Excellence. It will host meetings between scientists, government officials and agriculture-industry partners, who will be key to scaling up worldwide distribution of Salk Ideal Plants.
If you are interested in learning more about how you can make a difference in our work on climate change, contact Jane Rhett at (858) 453-4100 x1521 or jrhett@salk.edu. Climate change is a global problem that will require solutions from around the globe and participation by all of us.
To learn more about the Campaign for the Future, visit salk.edu/campaign.
Are you up for the challenge?
Featured Stories
The Salk Institute and Lustgarten Foundation form strategic pancreatic cancer research partnershipSupported by a $5 million grant, the partnership aims to identify and validate potential targets for new pancreatic cancer drugs. Four participating labs, led by Salk Professors Reuben Shaw, Ronald Evans, Tony Hunter and Assistant Professor Dannielle Engle, will bring their individual areas of complementary expertise to bear on the collaborative goal.
The weed that changed the worldHow Arabidopsis thaliana became one of the most important tools in science—and how the information the small weed has revealed over decades of research now enables the development of Salk Ideal PlantsTM, a new generation of food crops that are better equipped to both thrive in a changing climate and help mitigate it.
Courtney Glavis-Bloom — Shining the spotlight on aging to find a cure for Alzheimer’s diseaseSenior Staff Scientist Courtney Glavis-Bloom’s work is driven by her experience caring for her grandparents, who were diagnosed with Alzheimer’s disease when she was in high school. She saw firsthand how dementia robs individuals of their connections to the world—now she studies the brain areas affected in aging in the hope of finding a cure.
Helen McRae — Leveraging the body’s own immune response for more effective cancer therapiesHelen McRae was a graduate student when her cousin was diagnosed with lung cancer and received immunotherapy—an approach that empowers a patient’s own immune system to destroy tumors. McRae saw the promise of this newer treatment, but also how much more research is needed to help it work for more people.
Kenta Asahina – Flying into the future of technology and innovationOriginally from Japan, Associate Professor Kenta Asahina grew up exploring nearby farmlands with his two brothers in search of insects and plants. Their dad enjoyed taking them to the mountains and national parks. These experiences inspired his interest in the natural world, and Asahina now studies how genetics impact fruit fly behavior.