FRONTIERS The immune system: a question of balance
The immune system is a powerful biological force—a liquid organ that permeates our bodies. Diverse immune cells are constantly on patrol, hunting for miscreants to roust: bacteria, viruses, tumors, cellular trash. The immune system keeps us safe in a hostile world.
The Salk Institute is the house that immunology built. Jonas Salk’s groundbreaking work on the first polio vaccine propelled him to national attention and helped create a world-class research institute in Southern California. Even as the Institute has branched out into cancer, neurology and other fields, we still remember our roots.
And those roots run deep. The immune system is a powerful biological force—a liquid organ that permeates our bodies. Diverse immune cells are constantly on patrol, hunting for miscreants to roust: bacteria, viruses, tumors, cellular trash. The immune system keeps us safe in a hostile world.
“The immune system plays a profound role in maintaining human health,” says Martin Hetzer, a professor in the Molecular and Cell Biology Laboratory and Salk’s chief science officer. “Most of the time, it works so effectively, we don’t even realize how much we rely on it. But if we look at pandemics or rare immune conditions, like severe combined immunodeficiency (‘Bubble Boy’ syndrome), we realize how vulnerable we can be when our immune system is overwhelmed.”
But not every immune component is poised to attack. Some cells control the response, keeping it from running amok and attacking our own systems. These checks and balances are critical to healthy immunity. If the response is too weak, a pathogen or cancer can take hold. If it’s too strong, autoimmune conditions such as lupus, rheumatoid arthritis or multiple sclerosis can cause havoc.
Scientists call this careful balancing act between biological counterforces homeostasis, and researchers at the Salk Institute’s NOMIS Center for Immunobiology and Microbial Pathogenesis are working to delineate the mechanisms that make it possible. As the NOMIS Center celebrates its 10th anniversary this year, its researchers are answering many questions to illuminate immune function. How do different types of T cells respond to their environment, form memory and regulate immunity? Which mechanisms go awry in autoimmune diseases? Can we control infectious diseases without killing microbes?
Multidisciplinary researchers Susan Kaech, Björn Lillemeier, Ye Zheng, Janelle Ayres, Greg Lemke and others are investigating new ways to prime the immune system to attack disease or bring overactive cells under control. The goal? Restore balance.
UNDERSTANDING THE PLAYERS
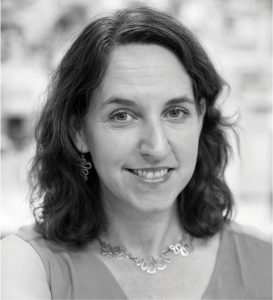
Susan Kaech
Immune cells are segregated into two major types: innate and adaptive. Innate cells are the first responders. They recognize a threat, mount an attack and call for help. Soon, the adaptive response kicks in. These cells are custom-designed to meet specific threats. Even better, the adaptive response remembers pathogens from years before, the mechanism that powers vaccines.
While accurate, that description vastly oversimplifies immunity. Scientists are still delineating the many signals that kick the immune system into high gear or keep it from overreacting. Even the number and types of immune cells are being questioned. For example, T cells are among adaptive immunity’s most prominent weapons, but it’s only recently that researchers have learned how varied they can be.
“Now we know there are subsets within subsets within subsets of T cells,” says Susan Kaech, professor and director of the NOMIS Center and holder of the NOMIS Foundation Chair. “The T cell population, as a whole, is being shaped by the inflammation that’s in the environment. That can dictate the types that form.”
Understanding this diversity is crucial on many levels. The immune response often weakens with age. Does this reflect fewer immune cells, a loss of diversity or other factors? Similar questions come up with cancer.
“We are investigating what central types of T cells, or functional traits they express, are critical to the anti-tumor immune response,” says Kaech. “We already know of some, but we want to figure out how we can orient therapies to produce and enhance that type of T cell to better enable the immune system to fight cancer.”
On a broader scale, Kaech and colleagues are exploring how different immune and non-immune cells communicate. This constant “cross-talk” is similar to pilots and air traffic controllers in busy air space. Immune cells alert other immune cells to potential danger. Normal tissue tells the immune system it is not dangerous. Tumors and pathogens try to fool immune cells into not responding at all.
Understanding these various inputs could offer new tools to control immunity. For example, like people, immune cells may not function optimally when they are hungry. Kaech is investigating how diseases, like cancer, could be “starving” T cells to limit their responses.
“Another level of cross-talk could be how the environment alters the availability of different metabolites and nutrients,” says Kaech. “How does this regulate immune cell function in cancer or infection? How might the metabolic state of particular non-immune cell types affect nutrient availability for the immune cells, and how does that in turn affect their metabolic state and functionality?”
This could be especially important in tumor microenvironments, which can be nutritional deserts. Kaech wants to understand, and ultimately control, these mechanisms to boost the immune response against cancer.
DECODING INTERNAL CHIT CHAT
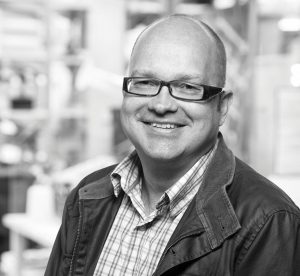
Bjorn Lillemeier
Immune research has been focused on external factors—the pathogens and molecular signals that kick the immune response into high gear. But what about internal signaling? What’s happening inside immune cells that spurs them into action?
There are two ways to investigate this. The classic approach studies which proteins talk to each other, a game of telephone that often extends to a cell’s DNA. But there’s also the spatial/temporal approach. How are these molecules in the right place at the right time to even have these conversations? Associate Professor Björn Lillemeier studies both.
“We are interested in how T cell receptors, which can sense a single molecule, are actually activating the entire T cell and inducing functional programs that are different between various T cell types,” says Lillemeier.
The system isn’t random—there’s no way T cells could respond fast enough. So how do key signaling proteins know where to be and when? These are difficult questions to answer because the scales are so small. The molecules the lab is tracking are around 2 nanometers and roam an area between 50 and 200 nanometers—a space 500 times smaller than the width of a human hair.
Traditional light microscopy can visualize objects as small as 250 to 300 nanometers, hardly suitable for such high-resolution studies.
If researchers try to image a 300-nanometer area, light sources that come too close together (in this case proteins) blur into indistinguishable blobs, like seeing a large city from space at night. The lab solved this problem by imaging individual proteins. The lab has embraced leading-edge super-resolution microscopy, which allows them to image areas as small as 25 nanometers. They take thousands of images to painstakingly piece together the larger picture. Lillemeier combines this approach with traditional biochemistry and cell biology, mutating proteins to see how the changes alter T cell function.
These efforts could have a major impact. Normal tissue turns on a T cell protein called PD-1 to counterbalance the activation of T cells. Tumors use the same trick to avoid immune attack. Cancer immunotherapies called checkpoint inhibitors turn off the enzyme to take the brakes off T cells and coax them to attack tumors. But this is a binary approach and only works for around 20 percent of patients with specific cancers, such as lung and melanoma.
Lillemeier’s lab has begun studying PD-1 to better understand how the proteins in this circuit move and interact. Eventually, he wants to provide more nuanced strategies to influence T cells. Being able to exert such precise control could open more patients to immunotherapies and mitigate side effects.
“These mechanisms involving PD-1 can be potential drug targets,” says Lillemeier. “Instead of targeting the activity of an enzyme, we can target where it is or how likely it is to be in a particular place.”
TAKING OUT THE TRASH TO DETER INFLAMMATION
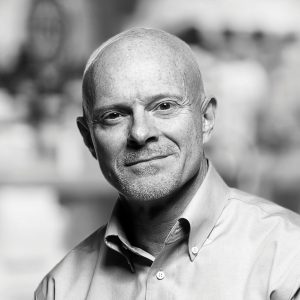
Greg Lemke
Greg Lemke, a professor in the Molecular Neurobiology Laboratory, studies TAM receptors (Tyro3, Axl, and Mer), which are found on macrophages and other cells and shut down an immune response after it has completed its work. But, as Lemke and others have shown over the years, that’s just the beginning of their responsibilities.
TAM receptors are also in charge of detecting dead cells. Millions of cells die each second—more than a hundred billion a day. It’s a normal process, but macrophages have to clean up the mess. With reduced TAM signaling, they stop engulfing dead cells, kind of like a garbage strike, causing a cascade of problems. It starts with chronic inflammation. Then, because the immune system stays ramped up, the body can slip into an autoimmune response.
“That’s bad because these dead cells will present antigens to the immune system, and the system will start making antibodies, and many of these antibodies are autoreactive—they attack the
body’s own organs,” says Lemke. “Diminished TAM signaling leads to autoimmune disease.”
The Lemke lab has shown that TAM signaling is a lot more important than previously thought. Just as tumors can turn off T cells, influenza, West Nile and other viruses can activate TAM receptors to evade immune surveillance. The lab also found that losing the Mer receptor in retinal cells can lead to blindness.
Dysfunctional TAM signaling also generates adverse consequences for microglia, the macrophages in the central nervous system. Without TAM-directed signals, microglia also stop disposing of dead cells, which contributes to, and exacerbates, neurodegenerative disease.
“Many of the genetic variables that increase the risk of developing Alzheimer’s disease turn out to be in and around genes that are only expressed in microglia,” says Lemke. “It’s clear that the regulatory functions of microglia—including controlling inflammation and engulfment—are important in Alzheimer’s.”
THE CURSE OF AUTOIMMUNITY
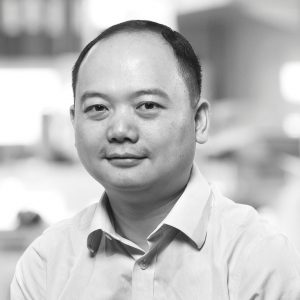
Ye Zheng
TAM receptors are one of many mechanisms designed to modulate the immune system. Tregs, which keep T cells from overreacting, are another way to dial down the immune response. In some ways, they are a firewall against autoimmune disease.
“Tregs are quite unique,” says Associate Professor Ye Zheng. “Most of the immune cells we know are designed to attack, to kill bacteria or viruses or whatever. Tregs suppress other immune cells from attacking.”
Tregs make up around 10 percent of all T cells and could hold the key to immune system homeostasis. Making them more suppressive could help researchers address autoimmune conditions. Making them less suppressive could promote the immune response against cancer.
For Zheng, the quest to better understand them begins with a protein called Foxp3, which is essential for Treg development.
“Some people have a mutation in Foxp3 and experience a massive autoimmune response called IPEX syndrome,” says Zheng. “Without the Tregs to suppress other immune cells, the body’s system becomes totally imbalanced and starts attacking everything.”
The Zheng lab has been focused on understanding the role Foxp3 plays in Treg development. That means identifying hundreds of interrelated genes—the ones that control Foxp3 and the ones that it controls. Through this detailed process, the lab has identified anomalies that can transform Tregs into cytotoxic T cells, generating autoimmunity in animal models. Zheng believes these and other findings could help lead to Treg-based therapies.
“If we can modulate Tregs, we could potentially take more precise control over the immune response,” says Zheng. “We could increase it to mitigate an autoimmune condition or decrease it to fight cancer. It could be a very powerful tool.”
WHAT BACTERIA CAN TEACH US
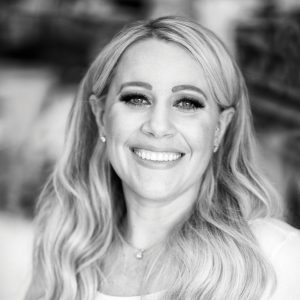
Janelle Ayres
Learning how various immune cells respond to pathogens—both individually and collaboratively—could give us better tools to manage infections. But the body has other mechanisms to deal with hostile microbes and the damage they cause. Associate Professor Janelle Ayres has a unique way to learn about these systems—by querying the pathogens themselves.
“The body has defense mechanisms that mitigate the damage associated with an infection,” says Ayres. “We focus on how microbes have evolved ways to induce these mechanisms themselves. We’re finding that microbial recognition by our immune system, to a large degree, drives these responses.”
Ayres is looking for alternatives to vaccines and antibiotics. It’s not that she’s against them, she just recognizes their limitations. Vaccines are not available for all viruses, and bacteria are developing resistance to our most powerful therapies. While many people believe the solution is new antibiotics, evolution is an unstoppable force. Sooner or later, microbes will develop resistance to the latest penicillin reboot.
On the other hand, what if evolution could be our friend? Around ten years ago, Ayres discovered the cooperative defense system, a collection of mechanisms that prevent physiological damage during infection. The system promotes health without killing the microbe and, in fact, is often initiated by microbes themselves.
A good example is sickness-associated anorexia (SAA), during which patients don’t feel the need to eat. The lack of nutrients can make infections like Salmonella more virulent, but that’s not a great outcome for bacteria. They need to keep their host healthy enough to keep hosting. But Salmonella has its ways. By blocking inflammation in the intestines, Salmonella prevents SAA and keeps its host alive.
“Salmonella has found a way to block this response from happening,” says Ayres. “It stays in the intestine, causes less virulent disease and lives to transfer to a new host. We can use microbes to teach us how to promote health because they’ve already figured it out.”
Rather than killing the pathogens, Ayres believes we can exploit cooperative defense mechanisms to protect people from collateral damage from Salmonella, sepsis and other conditions. Limiting the damage could give patients’ immune systems the opportunity to take care of the pathogen without pushing microbes
to evolve.
“We predict our approach will not drive drug resistance because we are targeting the physiology, rather than killing the pathogen,” says Ayres. “They have no reason to evolve around it.”
All encompassing
Because the immune system is everywhere in our bodies, NOMIS Center scientists collaborate on efforts throughout Salk’s many research areas: cancer, neurobiology, gene expression, cell biology, aging, metabolism.
Successful cancer immunotherapies are lighting the way for researchers to really deconstruct immunity. This work will eventually lead to new treatments for cancer, infectious diseases and autoimmune conditions, but it also illustrates how biology functions on the deepest levels.
“The immune system is a liquid tissue,” says Kaech. “It’s one of the few systems in our bodies where the cells go out and infiltrate all the other tissues. Thus, our immune system has the potential to interact with every cell type in our body and intersects with virtually every biological process. That makes it an important crossroad in science and an incredible opportunity for collaboration.”
Support a legacy where cures begin.
Featured Stories
The immune system: a question of balanceThe immune system is a powerful biological force—a liquid organ that permeates our bodies. Diverse immune cells are constantly on patrol, hunting for miscreants to roust: bacteria, viruses, tumors, cellular trash. The immune system keeps us safe in a hostile world...
Rusty GageComplacency has never been part of Fred (Rusty) Gage’s genetic make-up, neither as he has ascended to the ranks of the world’s most renowned neuroscientists nor as he’s taken the helm as President of the Salk Institute...
Emily ManoogianWhen Emily Manoogian was growing up, “scientist” wasn’t even on her list of potential careers. First Manoogian wanted to be a Broadway tap dancer; then a gymnast; later, a lawyer; and finally, towards the end of high school, a veterinarian.