Left photo: (from left) Wolfgang Busch, Diana Hargreaves, Nicola Allen, Sreekanth Chalasani and Saket Navlakha
OBSERVATIONS SALK’S NEW EXPLORERS A close-up of some of Salk’s junior faculty
Right photo: (from left) Renato Dubecco, Francis Crick, Edwin Lennox, Jacques Monod, Jonas Salk, Leslie Orgel, Jacob Bronowski and Melvin Cohn
Observations
Like people, institutions move forward generation by generation. The Salk Institute’s first group of scientists included founder Jonas Salk, famous for developing the first effective and safe polio vaccine; and Renato Dulbecco, who demonstrated how viruses can cause cancer and who was awarded the Nobel Prize in Physiology or Medicine in 1975.
This group imbued the Institute with a spirit of self-sufficiency. There were no departments, and faculty were expected to ask difficult questions and develop innovative ways to find the answers. But they were also part of a greater whole. An immunologist might have a lab next to a chemist who was, in turn, next to a cancer biologist. Like the cells they studied, these researchers were independent but interrelated.
Later, a new generation arose at the Salk Institute. To name just a few, Professor Ronald Evans identified the roles hormones play in cancer, diabetes and other conditions. Tony Hunter, American Cancer Society Professor, helped illuminate cancer-signaling molecules called tyrosine kinases, paving the way for targeted tumor therapies, such as Gleevec. Professor Joanne Chory pioneered efforts to understand how plants sense light and produce growth hormones, knowledge that could help increase food supplies by growing plants adapted to harsh environments and a changing climate. And Ursula Bellugi, distinguished professor emerita, illuminated how the brain processes American Sign Language and other human interactions, shedding new light on neural function.
And now, another generation of Salk researchers is just as brilliant and driven as its predecessors. These scientists are asking bold questions about cancer, neurons, plant biology and the immune system. They look for support and inspiration from their peers in other disciplines, and they’re not afraid to take risks to figure things out. Inside Salk sat down with several junior faculty across a sampling of fields to see how they are tackling pressing biological problems and what inspires them.
NICOLA ALLEN
ASSISTANT PROFESSOR
MOLECULAR NEUROBIOLOGY LABORATORY
HEARST FOUNDATION DEVELOPMENT CHAIR
Why did you choose Salk?
I’m a neuroscientist, so my other choice would have been to go into a neuroscience department, which would also have been fantastic, but then you’re interacting with people who think about the same questions as you all the time.
Here, I have to explain what I’m doing to people who work in plant biology or cancer biology, and they can come back with novel insights. I like being in a place where people have broad research backgrounds because it helps me think about my science in a different way. That back-and-forth really expands the research.
What big questions are you trying to answer?
The big question for us is: How does the brain actually form? The human brain has billions of neurons, and they have to find the right partner during development and connect at a place called a synapse, and there are trillions of these.
We want to understand how this happens and how it goes wrong. There are a lot of neurodevelopmental disorders, such as autism spectrum disorders and schizophrenia, where there’s some level of disconnection. We want to understand if we can fix it when it gets damaged.
Compared with others in your field, how is your approach different?
A lot of neuroscientists are interested in these questions. The way we approach it is to think, okay, we know the brain has lots of neurons, but we also know they’re surrounded by other cells, called glia. We’ve been working to understand how these glial cells, specifically astrocytes, talk to neurons and regulate their function. They are the cells telling neurons when to make connections and how to change those connections with experience. We believe we can use this information to repair these connections when they’ve been damaged.
How have technological advances impacted your work?
We’re not driving technology, but we’re always on the lookout for technological advances, like improvements in sequencing. Also, massive data sets are being generated by other researchers who are looking at these glial cells. So now we can use that data to look at our favorite genes, which we know could be involved in different disorders, dig into it and see what’s going on.
JANELLE AYRES
ASSOCIATE PROFESSOR
NOMIS CENTER FOR IMMUNOBIOLOGY AND MICROBIAL PATHOGENESIS
HELEN MCLORAINE DEVELOPMENTAL CHAIR
What are the big questions you’re trying to answer?
The traditional perspective for treating infectious diseases is to kill the pathogen, and that view has really shaped how immunological research is done. We have an excellent understanding of how the immune system kills infectious disease microbes. I felt that view was too simplistic: there must be other things that ensure people survive infections.
For example, sepsis is caused by microbes entering parts of the body that are otherwise sterile. When our immune systems recognize these microbes, they mount an inflammatory response that is so powerful it can lead to multi-organ failure and death.
The traditional way to treat sepsis is to give patients broad-spectrum antibiotics or antivirals, but it’s the patient’s own response that’s doing more damage than the microbe itself. We have to find ways to fix that damage to ensure the patient is going to survive.
My lab discovered that our bodies encode mechanisms we call the cooperative defense system, which protects us from infectious diseases by alleviating physiological damage without killing pathogens. We’re trying to understand the underlying mechanisms of the cooperative defense system so we can promote patient survival without killing microbes.
How do you, as a foundational scientist, see the impact of your work?
The problem is, society has been focusing entirely on new ways to kill microbes—it’s our sole approach against infectious diseases. But antibiotics drive microbial resistance, and we haven’t developed vaccines for many infections. We want to develop evolution-proof drugs to treat infectious diseases that won’t drive drug resistance.
We’re on the right track with that. We can boost the cooperative defense system, and we can treat infections without microbes developing resistance. Also, by promoting the cooperative defense system, we can make pathogens evolve into benign microbes that no longer cause disease. We’ve shown that can happen.
Which Salk scientists have inspired you?
I think a lot of people, after they’ve had their labs for 30 or 40 years, slow down a bit. But some scientists, like Ronald Evans, show no signs of slowing down. He’s not scared to go in new directions. He will look at a field, see there’s a problem, find a way to approach that problem, and go for it. That is inspiring.
What job would you have in an alternate universe?
If I had another job, it would involve rescuing animals. I am always saddened by the number of stray dogs I see when I visit other countries, particularly in Central and South America. I want to somehow help those animals and don’t want to wait to be in an alternative universe to do it.
SREEKANTH CHALASANI
ASSOCIATE PROFESSOR
MOLECULAR NEUROBIOLOGY LABORATORY
Why did you choose Salk?
I thought, and I still think, that Salk is the best place for me because it creates an environment where I can do absolutely crazy science, in the sense that I can take the science in directions I could not even dream of at other places. I came in knowing that, within walking distance, there’s an expert in protein chemistry, in mathematics, and in bioinformatics, for example.
What big questions are you trying to answer?
Experience is called different things in different contexts. Sometimes it’s called learning. But if a prior experience was stressful, it might cause fear and generate an anxiety response later in life.
We’re trying to understand the fundamentals of how the nervous system extracts information from the environment and integrates current information with past experience to generate an appropriate response. Understanding these basics could help us to better treat conditions such as post-traumatic stress disorder, anxiety disorder and autism.
There are 86 billion neurons and 150 billion non-neuronal cells in the human brain. That’s more connections than there are stars we can see. It’s overwhelming, so we use simple systems to solve these complex problems. In particular, we study worms called C. elegans, which have just 300 neurons and 9,000 connections. C. elegans can learn and remember, and show fear and anxiety in circuits that have similarities to mammals. They are powerful tools to understand how the brain works.
How do you, as a foundational scientist, see the impact of your work?
The most common thing people have observed in children with an autism spectrum disorder is reduced social interaction. By sequencing these children’s DNA, researchers have identified changes in about 800 genes, compared to children with typical development. If I take one of those genes and mutate it in C. elegans, that worm will not join a social aggregate; it just hangs out by itself.
I’m not saying the C. elegans has autism, just that nature uses the same mechanisms to solve the same problems across the plant and animal world. That’s why simple systems can work so well to help us understand larger, more complex systems like the human brain.
What do you hope to accomplish in the next 10 years?
For questions as complex as those my team and I are working on, we needed to develop new technology. We use ultrasound to manipulate cells noninvasively in a new area I coined “sonogenetics.” This interdisciplinary program is unique to Salk.
We believe our technology can replace many surgical interventions. For example, we want to embrace deep brain stimulation, which is used to treat Parkinson’s disease. We want to build a biological pacemaker that could replace an electronic pacemaker. We want to replace an electronic insulin pump with a biological pump. And we are optimistic we can get there.
DIANA HARGREAVES
ASSISTANT PROFESSOR
MOLECULAR AND CELL BIOLOGY LABORATORY
RICHARD HEYMAN AND ANNE DAIGLE ENDOWED DEVELOPMENTAL CHAIR
Why did you choose Salk?
I knew about the science at Salk and how expansive it is. When visiting, I was explicitly told by Tony Hunter that they expected me to be doing something different in five years, to be collaborating, to draw from my neighboring labs and allow them to push me to venture into areas I normally would not go.
The Institute’s structure facilitates these interactions. There’s a community spirit, a sense we are here to cross interdisciplinary boundaries. It’s not just talk. I can see it happening in the research.
What big questions are you trying to answer?
We are interested in epigenetic regulators. These are proteins that largely tell a cell which genes to turn on and which ones to turn off. They are highly mutated in cancer. Our goal is to understand how these mutations disrupt cellular function and ultimately give rise to disease.
Compared with others in your field, how is your approach different?
We started in a relatively straightforward way, just looking at the mutations. But our data led us to start thinking about non-epigenetic processes. We’re getting interested in interactions with mitochondria and other organelles that, normally, I would not be studying at all. It’s the collaborations here that allow us to do that.
For example, we’ve started collaborating with Salk Professor Gerald Shadel. Some of the findings in our data were similar to findings his team had, from manipulating mitochondria. We looked at each other and he asked, “Do you think mitochondria are affecting the nucleus, or do you think genes in the nucleus are affecting mitochondria?”
Maybe we can look at his signature in my cells, and my signature in his cells, and get down to how these two organelles are communicating. It’s a nice example of this collaborative spirit.
How do you, as a foundational scientist, see the impact of your work?
The impact is fairly direct. We know these mutations are highly prevalent in cancer. What we don’t understand is why. We have evidence in the human population that they are definitely cancer drivers. We just don’t have a sense, mechanistically, of how that is happening.
We’re talking about common cancers: colorectal, lung, ovarian. That resonates with family and friends because many people actually have cancer or know someone who has cancer.
What inspires you?
My lab inspires me. The data inspires me. I really enjoy being at Salk. I find it incredibly inspirational looking at my colleagues and what they’re coming up with.
SAKET NAVLAKHA
ASSISTANT PROFESSOR
INTEGRATIVE BIOLOGY LABORATORY
PIONEER FUND DEVELOPMENTAL CHAIR
Why did you come to Salk?
It was definitely not part of the plan. My background is in computer science. I spent pretty much all of my undergraduate, graduate and postdoc years in computer science or machine learning. That was home for a long time.
My interest in biology started during my postdoc. It connects to the theme of my lab now, which studies algorithms in nature—this idea that biological systems have to solve problems to survive. These are basic computational problems that arise and, over millions of years of evolution, some of these systems have evolved really ingenious strategies to solve them. It’s amazing what millions of years of randomness and selection can do. We want to learn the strategies these systems have evolved in order to enhance computer science and machine learning, as well as present a new perspective on how biological systems process information.
As I started applying to organizations, I was much more excited talking to someone who was doing immunology or plant biology or cancer biology than I was talking to computer science people studying computer graphics. The idea of having colleagues who have in-depth knowledge of many different biological mechanisms, that’s exciting for me.
What big questions are you trying to answer?
One area I’ve been studying is the interface between machine learning and neuroscience. The brain is one of the most efficient computational devices in the world. It’s doing things that no computer can do, and it’s doing it with approximately 20 watts of power, which is like a standard light bulb. So the dream is to understand the basic principles of how the brain works in such a way that we can use these strategies to enhance machine learning.
We’re in a time now when we can really map out circuits and connectivity, electro-physiology and anatomy, in ways that get into the details of how the brain actually works.
What job would you have in an alternate universe?
When I was growing up, I thought I’d be playing in the NBA. I thought I’d be the first Indian playing for the Miami Heat. I’d be draining threes from the corner, and the crowd would be going wild. I believe in the multiverse. In some other universe, that’s what I’m doing.
WOLFGANG BUSCH
ASSOCIATE PROFESSOR
PLANT MOLECULAR AND CELLULAR BIOLOGY LABORATORY
INTEGRATIVE BIOLOGY LABORATORY
How do you, as a foundational scientist, see the impact of your work?
The key question in genetics asks how an organism’s characteristics (its phenotype) are encoded into the genome. Which parts of the genome, and which mechanisms, make us different from animals or animals different from plants? To study this, I use root systems.
Trees take a long time to grow. We want to use the genome sequence, when it’s a seedling or even a seed, to determine what a tree would look like and whether it would withstand certain environmental conditions, such as stress and disease.
Roots search the soil for nutrients and water, but not all nutrients are distributed equally. If we understood which genes help roots find nutrients, we could tailor crops for specific soils or environmental conditions.
This is even more important now with climate change. If I knew which genes were important, I could tell farmers they need to plant this varietal to get the maximum output from this soil in these conditions. We could tell breeders to cross certain varietals or we could introduce gene editing to make a new subtype that is well-adapted and maximizes output.
How have technological advances impacted your work?
We measure many different strains and identify the genes responsible for root development. We use a method called genome-wide association mapping, based on having the sequence information of many varieties. That has only become possible over the past 10 years thanks to the sequencing revolution.
We can also measure a lot of phenotypes, such as root length or thickness. Image-processing software is essential for that, and we are using deep learning to increase our abilities. We do a lot of measurement, so advances in the ability to compute complex statistics have really driven our work forward.
We pioneered tools to grow a lot of roots, take pictures and measure the roots from these images. Initially, we measured around three million to four million roots. Since coming to Salk, we have built a robot that allows us to screen millions of plants each year.
Which scientists inspire you?
There’s a long list, but Charles Darwin and Gregor Mendel are the giants. Darwin even experimented with roots, and postulated that root tips act as a kind of brain for the plant.
At Salk, it’s Joanne Chory and Joe Ecker. Even when I was still a student, I knew of Salk because the first reverse-mutant collection [mutated genes that are further altered to enhance study] was made here by Joe Ecker. Joanne Chory did fundamental work on how plants sense the environment and respond to it, and she investigated the mechanisms that are responsible. Salk was always on my radar.
SALK’S UP-AND-COMING SCIENTISTS
The Helmsley-Salk Fellows Program brings impressive young scientists to the Institute to drive innovation. Having recently earned their doctorates, these investigators embrace original ideas and novel approaches. In other words, they fit right in.
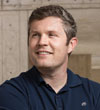
Jesse Dixon, who received his MD/PhD from UC San Diego, studies chromosomes and how their overall structure governs gene expression and genome organization. His work aims to understand cancer, evolution and other processes.
Many researchers study how different molecules (proteins, RNAs, etc.) control gene expression. Dixon is looking at the forest rather than the trees. He wants to understand how genomes are organized in three-dimensional space, how that packaging regulates gene expression and how it might go awry in disease.
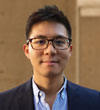
Patrick Hsu earned his PhD at Harvard and was an early contributor to CRISPR-Cas9 gene-editing technology. He wants to refine this technology to improve patient care. Hsu combines synthetic biology, genomics and neuroscience to address neurodegenerative diseases and complex genetic disorders.
He uses gene editing to examine cell processes and understand how small genomic adjustments can generate big changes in an organism. In addition, Hsu’s lab has developed a new CRISPR system to edit RNA, which will help him and others gain even more insight into how the genome mediates health and disease.
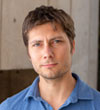
Dmitry Lyumkis was recently promoted to assistant professor. He was one of the first Helmsley-Salk Fellows, coming to the Institute in 2014. The following year, he was honored with a prestigious NIH Director’s Early Independence Award.
Lyumkis is trying to solve one of the most difficult problems in biology—how to image a protein to discern its structure. This is critically important work because, with proteins, structure equals function.
To get there, Lyumkis uses an emerging electron microscopy technology called cryo-EM, which allows him to visualize proteins in near-native conditions and build three-dimensional models.
Lyumkis is particularly focused on illuminating the atomic machinery that helps HIV insert its DNA into human genomes. This structural information could lead to new therapeutic targets and antiviral drugs. His lab is also refining the technology to achieve higher resolutions and image other biological machines, such as ribosomes, which read RNA and build proteins.
Support a legacy where cures begin.
Featured Stories
Salk’s New ExplorersLike people, institutions move forward generation by generation. The Salk Institute’s first group of scientists included founder Jonas Salk, famous for developing the first effective and safe polio vaccine; and Renato Dulbecco, who demonstrated how viruses can cause cancer and who was awarded the Nobel Prize in Physiology or Medicine in 1975.
A matter of timeSalk Professor Satchidananda (Satchin) Panda runs his life like clockwork. Most mornings, if he’s not traveling, he wakes up around 6 a.m. without an alarm. One of the first things he does is go out to his backyard to check on his provisions for wild birds.
Driven to SucceedFrom once being a schoolboy sitting on the floor of a rural classroom with no electricity, to now being a breast cancer researcher in the laboratory of Geoffrey Wahl, Raj Giraddi’s deep and abiding interest in biological research has always driven him forward.